Narrowing the metabolic engineering divide between plants and microorganisms will require faster design-build-test cycles and better genome engineering tools.
Plants are the ultimate source of foods, fibers, pharmaceuticals, and fuels. This article discusses the metabolic engineering of plants to produce fuels and value-added chemicals, with a focus on the general concepts and challenges that differentiate plant and microbial engineering. Two examples of successful metabolic engineering of plant traits are highlighted, and emerging techniques to prevent transgene release into the wild and to advance the speed of the design-build-test cycle are discussed.
Conversion schemes for commodity chemicals and fuels from plant biomass
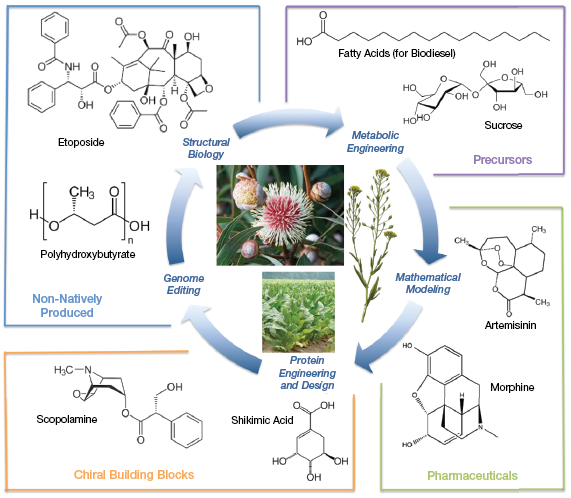
▲Figure 1. Many different chemicals and fuels can be produced from plants; the photos in the center are several representative species. The outer boxes show various chemicals that can be produced from plants, including shikimic acid and morphine. The inner ring shows different enabling technologies that facilitate production routes for these and other fuels and chemicals.
Plant biomass is an output of carbon dioxide, sunlight, water, and soil-derived nutrients. This biomass can be used directly or indirectly for fuels and value-added chemicals (Figure 1).
For thousands of years, pharmacologically active molecules have been extracted from plants, including opioids from poppies and antimalarial medicines such as artemisinin from Chinese wormwood. Today, chiral building blocks are sourced from plants for a variety of pharmaceuticals. For example, shikimic acid is produced in anise seeds for the synthesis of the antiviral oseltamivir (marketed as Tamiflu) (1) and scopolamine is extracted from corkwood leaves for synthesis of various bronchodilators (most notably tiotropium, which is marketed as Spiriva) (2).
Plant biomass can be converted to biofuel using physicochemical and/or enzymatic treatments to break it down into sugars, followed by upgrading via fermentation or chemical catalysis. Diesel and jet fuel can be prepared from seed oils from palm trees or plants like Camelina sativa. Finally, fast-growing plants like tobacco can be genetically engineered to accumulate value-added chemicals (3) and drugs (4). Thus, there is a wide diversity of feedstocks and speciality chemicals that originate in plants.
Comparing products made by engineered plants and microbes
Not every chemical can be produced by plants. One major issue is volatility: many plant-produced volatile compounds leak into the atmosphere, making collection difficult. Take, for example, the C5 monomer isoprene, which is used in rubber. Although trees and other plants emit over 500 million tons of isoprene annually, little is left in plant biomass (5). It is much easier to recover volatiles for downstream purification from off-gases in closed fermentation vessels. To that end, Danisco, in collaboration with Goodyear, has developed a bioprocess for production of isoprene using metabolically engineered E. coli (6). Researchers have implemented several strategies to mitigate the escape of plant-produced volatiles, including adding a protecting group or handle to keep the molecule inside of the plant (7) and redirecting biosynthesis to plant subcompartments.
Another issue is compound toxicity that can limit plant yields. This is the case for classical fermentation products — such as citric acid and ethanol — that can be produced by fermentation at near-quantitative yields and high product titers. Even though many plants naturally produce many of these compounds (e.g., citric acid in citrus fruits), the process of extracting and purifying these products from diffuse point sources is economically less favorable than fermentation using inexpensive sugar sources like beet molasses.
However, in many cases it is more desirable to produce biofuels or bioproducts directly in the host plant. Biodiesel is one such example. For example, the nonfood oilseed crop Camelina can yield two metric tons of biodiesel with approximately 40% by weight lipids per hectare. Seed lipids are trapped in oil bodies that can be recovered and hydrotreated to yield a renewable diesel that is interchangeable with petroleum-derived diesel. Alternatively, the recovered lipids can be subjected to catalytic cracking to alter the distribution of chain lengths to the appropriate range for jet fuel and gasoline. In general, products accumulate at high abundance if they are part of the reproductive strategy of the organism — oil accumulation in the seeds, sucrose in beets and sugarcane, and so forth.
A large engineering gap exists between plants and microbes
The plant metabolic engineer has many...
Would you like to access the complete CEP Article?
No problem. You just have to complete the following steps.
You have completed 0 of 2 steps.
-
Log in
You must be logged in to view this content. Log in now.
-
AIChE Membership
You must be an AIChE member to view this article. Join now.
Copyright Permissions
Would you like to reuse content from CEP Magazine? It’s easy to request permission to reuse content. Simply click here to connect instantly to licensing services, where you can choose from a list of options regarding how you would like to reuse the desired content and complete the transaction.