Hazardous-waste disposal presents unique challenges. An analysis of several incidents highlights the importance of properly characterizing waste prior to processing.
The chemical process industries (CPI) inevitably produce waste. Although minimization efforts are essential, waste is still generated and must be safely disposed. The chemicals involved in the front end of manufacturing processes are typically well-defined, including their hazardous properties and potential interactions. In contrast, waste streams are often ill-defined, which makes identifying hazards and preventing incidents challenging.
The CPI have increasingly adopted process safety management (PSM) principles for handling hazardous chemicals in the past decades. This cultural evolution has been driven, in part, by major accidents. PSM is more complex when applied to waste composed of unknown, mixed, and uncontrolled chemicals.
This article provides some background on waste-handling fundamentals and analyzes several fires and explosions at waste-handling facilities. Despite the significant differences among these incidents, they can all be attributed to a gap between the real and recognized properties of the wastes.
The basics
Title 29 Part 1910.119 of the U.S. Code of Federal Regulations (CFR) details process safety management principles for highly hazardous chemicals. The Center for Chemical Process Safety (CCPS) expands on this process safety model and defines 20 elements of risk-based process safety (1). It is good practice to apply these management principles to waste handling and treatment, which requires appropriately characterizing the waste. However, rather than holistically applying PSM principals, hazardous-waste handling facilities typically follow a prescriptive approach to characterize and handle waste based on U.S. Environmental Protection Agency (EPA) regulations, such as those under the Resource Conservation and Recovery Act (RCRA) (Title 40 CFR Parts 239–282).
The EPA reported that 26,237 operations produced 33.6 million tons of hazardous waste in 2015, of which, over 6 million tons were non-wastewater (2). Hazardous waste, as defined in RCRA, must be managed to meet a variety of criteria related to its final form and potential hazards. Determining the chemical properties of waste can be challenging, because streams might consist of separate phases or reacting or changing components, and might be ill-defined by the waste generator. Because it is difficult to identify the properties and hazards of waste streams, managing waste hazards is inherently more challenging than managing typical chemical process hazards.
Industrial waste may pass through a municipal waste transfer station en route to a landfill or be collected, aggregated, and incinerated by a permitted hazardous-waste treatment, storage, and disposal facility (TSDF), depending on the source, industry, and regulatory classification of the waste stream. Facilities may not be equipped to handle the complexity and unknown hazards of some wastes.
Evaluating chemical reactivity hazards. Hazardous waste may be flammable, corrosive, reactive, and/or toxic. The RCRA regulations (40 CFR Part 261) detail the criteria that define whether a waste is hazardous based on characteristics such as ignitability, corrosivity, and toxicity. The EPA does not currently provide a test method for assessing reactivity. Table 1 includes a partial list of criteria for characterizing hazardous wastes. There are many exceptions and details not fully covered in Table 1 that can be found in 40 CFR Part 261.
Table 1. The U.S. Environmental Protection Agency (EPA) provides criteria for characterizing hazardous waste. | ||
Characteristic | Criteria | Regulation |
Ignitability | Liquid with a flashpoint less than 60°C (140°F) Ignitable solid as determined by Method 1030 Oxidizing solid as determined by Method 1040 Spontaneously combustible as determined by Method 1050 | 40 CFR §261.21 |
Corrosivity | pH less than or equal to 2, or greater than or equal to 12.5, and/or corrosive to steel as determined by Method 1110A | 40 CFR §261.22 |
Reactivity | Unstable under normal conditions and/or reactive with water | 40 CFR §261.23 |
Toxicity | Toxic as defined by Method 1311 | 40 CFR §261.24 |
Facilities that generate hazardous waste are required to classify the waste before sending it to a TSDF. After it receives the waste, the TSDF must perform a preacceptance analysis to confirm the characteristics and identify incompatible wastes (3). Depending on the processes employed by the TSDF, chemicals may be mixed or packed together during processing or shipping. Chemicals that are incompatible might react and generate heat or produce flammable, toxic, or inert gases or mixtures that produce toxic substances, fires, or explosions.
According to the EPA, compatibility testing may include determining water reactivity (e.g., foaming, heat generation, explosivity), oxidation-reduction potential, and cyanide and sulfide content, among others. Waste compatibility can be evaluated by physical tests or based on known properties of each of the streams' constituents. If waste streams are being checked for compatibility using known information about the streams’ constituents, a certain level of confidence in the compositions of the waste streams is necessary to evaluate potential reaction consequences.
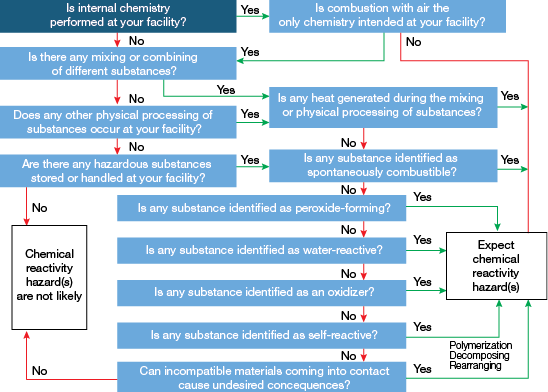
▲Figure 1. This decision tree can help you to determine if a chemical reactivity hazard is likely.
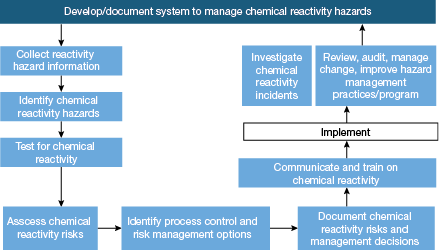
▲Figure 2. If a chemical reactivity hazard is likely, follow these steps to develop and document a system to manage the hazard.
CCPS published a preliminary screening approach based on a series of questions to help facilities decide if a chemical can pose a reactivity hazard (Figure 1) (4). Screening should be conducted on an ongoing basis, particularly if the process or processing conditions change or a new chemical is introduced into the source process. If a chemical reactivity hazard is expected, CCPS also suggests implementing a chemical reactivity management program (Figure 2).
Protocols for investigating reactive chemical incidents. Two resources for performing incident investigations are the National Fire Protection Agency (NFPA) 921 Guide for Fire and Explosion Investigations, 2017 edition, and the CCPS book Guidelines for Investigating Chemical Process Incidents, second edition (5, 6). These resources outline the scientific method, investigation techniques, and fundamentals of fires, explosions, and reactive chemical incidents.
Because fires and explosions, as well as the associated emergency response, often destroy essential evidence needed for an investigation, conclusively determining the chemistry and causes may be impossible. Thus, investigators must rely on the scientific method to develop hypothetical causes, test those causes, and determine the most likely causes and contributing factors.
Treatment, storage, and disposal facilities
The ultimate fate of hazardous materials is often destruction by incineration. TSDFs commonly repackage wastes, either by combining similar waste streams in the same container or by breaking up large containers into smaller containers. Thus, hazardous materials often pass through the following stages at a TSDF:
- receipt and characterization
- temporary storage
- repackaging, either bulking up or breaking down
- storage awaiting disposal
- final disposal.
TSDFs typically sample and perform basic characterization of wastes as they are received. However, this process is imperfect. It is not guaranteed that samples from distinct locations in a waste container represent the bulk of the waste in the container or shipment. The potential for deviations from actual properties increases significantly for larger containers (e.g., a 1-m3 box) and large volumes of wastes that are not completely sampled (e.g., only a subset of the containers are sampled), as well as wastes that are heterogeneous (e.g., layered, contain pockets of different compositions).
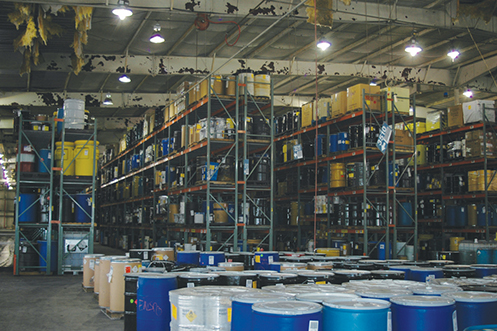
▲Figure 3. This treatment, storage, and disposal facility (TSDF) contains thousands of storage vessels.
Incident investigation at TSDFs can be very challenging. A TSDF storage area may contain thousands of individual waste containers (Figure 3). A fire or explosion in the storage area can destroy or damage the waste containers, displace containers, and mix residues from adjacent containers. This makes it difficult to determine the cause. The likelihood of determining the actual cause typically increases if the incipient stages of the fire or explosion are witnessed.
Fires and explosions in storage operations
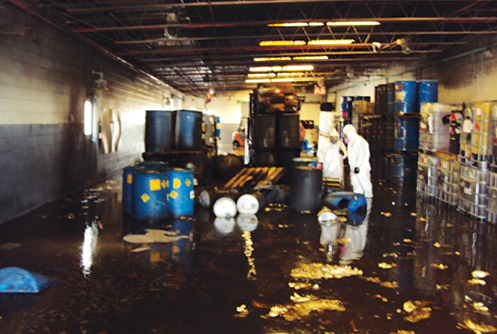
▲Figure 4. After a fire occurred in a storage bay, investigators were able to determine that the release came from the middle row of containers.
A fire occurred in a small TSDF storage bay. The facility handled a wide variety of wastes, including flammable materials and acids. The facility layout included storage bays, laboratories, treatment equipment, and office areas. Intermediate bulk containers (IBCs) and sets of four 55-gal drums were placed on wooden pallets and stacked two or three levels high in the storage bay (Figure 4).
According to witness statements, the fire in the storage bay was preceded by a loud hissing sound and a pressurized release of a reddish dust/mist from a container near the center row of the containers in Figure 4. The witness did not see the exact container from which the release originated, but was able to estimate its approximate location within a few pallet stacks. After the fire started, fire suppression efforts were able to contain the fire damage to the storage bay where it originated.
The investigation focused on the adjacent rows containing the waste drums that were damaged. Investigators reconstructed the exact location of each waste container in the neighboring pallet stacks based on storage records, remaining containers, damage patterns, and witness statements about container placement. They confirmed the origin to be one pallet stack in the center row.
The containers in the center row had not been repackaged or disturbed from the time of receipt until the incident. Therefore, the investigation could refute commingling of incompatible wastes or mixing of a reactive pocket within the source drum as potential causes.
Delayed decomposition of waste material in one of the drums was determined to be the likely source of the pressurization. The pressure eventually increased until the source container ruptured and the fire ensued. There was significant uncertainty about the exact contents of each container; thus, a specific container could not be identified as the origin of the release.
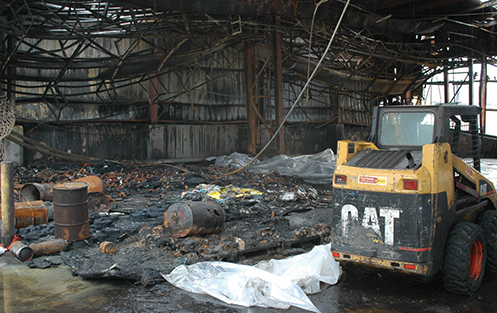
▲Figure 5. Despite extensive damage to this storage facility, investigators were able to pinpoint the origin of the release because of witness statements.
The extent of damage does not always preclude determination of the likely cause of a fire during storage. A similar TSDF had a fire in a storage bay that consumed all of the hazardous waste and combustible containers in the area (Figure 5), but investigators were able to precisely place the origin of the fire.
A nearby operator saw that the fire began at ground level at a specific pallet stack. The source of the fire was most likely a leak of fuming red nitric acid that spilled onto the wooden pallet holding the drums. Fuming nitric acid is a strong acid capable of igniting wood. The facility had not identified the potential consequences of an acid spill; thus, responsible parties did not select a noncombustible pallet material (e.g., steel).
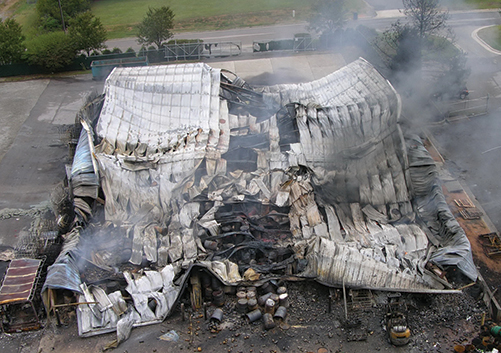
▲Figure 6. The release at this TSDF destroyed the facility and forced residents nearby to evacuate.
A fire at a different hazardous-waste TSDF destroyed the facility (Figure 6). The initial cause, however, could not be determined. The magnitude of the incident forced thousands of local residents to be evacuated over two days. The U.S. Chemical Safety Board (CSB) investigated the incident and identified several potential causes (7).
Witnesses reported a strong chlorine odor and smoke plume, and the first firefighters on the scene reported a small fire. The fire started in one of the hazardous-waste storage bays and rapidly spread to other locations in the facility, including a bay containing many 55-gal drums of flammable solvents. The incident investigation revealed that the fire originated in a storage bay where containers of solid chlorine-containing pool chemicals and unspent aircraft oxygen generators were awaiting final disposal.
Witness observations of a chlorine odor and smoke likely indicate that these chemicals were present near the fire origin. Investigators, however, were not able to definitively conclude that these chemicals were the cause of the fire due to the extent of the damage.
The CSB investigation findings focused on chemical oxygen generators (8). The CSB concluded that chemical oxygen generators most likely contributed to the intensification of the fire, which quickly spread to an adjacent storage bay of flammable materials. The CSB recommended that unspent oxygen generators should be actuated before transportation.
Many incidents have occurred involving storage of chlorine-containing pool chemicals (Refs. 9–12 offer examples).
Fires and explosions during incineration
Hazardous wastes with an intrinsic heat of combustion are often incinerated in furnaces, boiler combustion chambers, rotary kilns, or other combustion equipment as supplementary fuels. Fuel containers and liquid feeds may be mixed or provided in separate containers to achieve target average heats of combustion and emissions. The use of wastes as fuels has been associated with incidents outside of the actual combustion equipment.
An explosion occurred at an incineration facility that processed hazardous and nonhazardous solid and liquid wastes through thermal destruction in a rotary kiln. Drums of waste were homogenized in a shredder prior to being fed to the kiln. Due to the potentially flammable nature of the waste and the likelihood that the shredder would generate mechanical sparks and/or heat, the system was inerted with nitrogen gas. The nitrogen inerting function was performed over a fixed time period. The system automatically moved individual pallets of waste into an airlock, purged the airlock with nitrogen, and then dumped the pallets into the shredder chamber, which had already been inerted with nitrogen. After shredding, a screw conveyor moved the homogenized waste to the rotary kiln.
A central incinerator control room at the facility monitored several operating parameters, including the oxygen concentration and temperature within the inerted equipment. Since one of the primary safety mechanisms for the unit was a low-oxygen-concentration atmosphere, the shredder was not intended for oxidizing materials, such as solid oxidizers, oxygen containers/cylinders, explosives, and/or materials that react with water. During shredding of one waste load, an explosion occurred in the shredder chamber, which damaged the unit and opened explosion panels on the shredder chamber and airlock.
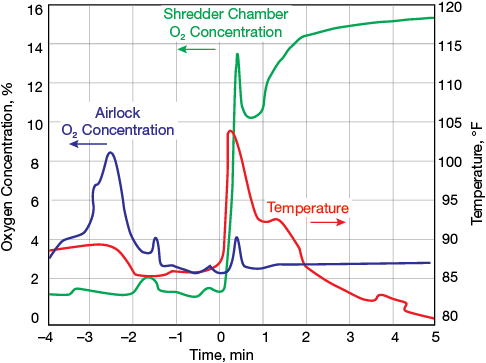
▲Figure 7. The temperature in the shredder chamber suddenly increased at approximately the same time as an increase in oxygen concentration, which suggests a combustion reaction occurred in the chamber.
Figure 7 shows oxygen concentration trends inside the airlock and shredder, as well as the temperature in the shredder chamber. On the night of the incident, a waste load was fed into the unit, indicated by the peak in oxygen at –2.5 min. After nitrogen purging, the load was transferred to the shredder and shredding commenced. Approximately one minute after shredding began, the oxygen concentration started to increase, from 2% to more than 13% over a period of 20 sec. According to the recorded data, the temperature in the shredder chamber suddenly increased at approximately the same time as the increase in oxygen concentration. This temperature increase is consistent with the heat generated by the combustion explosion in the shredder chamber. An engineering investigation concluded that the source of the rapid oxygen influx was an item in the waste stream that released oxygen when it was shredded, most likely a small pressurized cylinder. All potential sources of air via external sources were examined and rejected as causes.
The explosion in the shredding system demonstrates the serious consequences of processing waste with unintended properties. In this case, a system that was intended to operate while inerted inadvertently contained compressed oxygen, resulting in the explosion. Although the waste was screened before it was fed into the shredder, the screening process did not identify and divert the oxygen-containing waste. The explosion mitigation system functioned correctly by relieving the explosion pressure through explosion vents. The system was damaged, but the damage was not catastrophic, and there were no injuries.
Another incident involved the explosion of a tank car containing toluene diisocyanate (TDI) waste material that was used as supplementary fuel in a rotary kiln at a cement plant operating as a hazardous waste TSDF. The incident occurred when a rail tank car exploded in the unloading area and was propelled about 750 ft away (13–15). Other tank cars, as well as storage tanks located nearby, were also heavily damaged. No injuries or fatalities were reported; however, property loss was significant.
This facility received several tank cars containing column bottoms waste from a TDI manufacturing process. TDI is a monomer feedstock used to manufacture polyurethane polymers. The waste generator had stored tank cars containing this type of waste material for several years, and reprocessed them as the opportunity arose. Prior to the incident, several tank cars of TDI material had been shipped to the TSDF for incineration, and were successfully emptied and the contents incinerated in the kilns. The TDI waste was partially polymerized in most tank cars. The investigation determined, however, that the contents of the tank car involved in the incident were heterogeneous and their properties were not the same as those of the previous batches.
The facility used external steam heating via built-in steam jackets on the tank cars to reduce the viscosity of the polymerized TDI to enable transfer into the kilns. Two days before the incident, the facility made three unsuccessful attempts to unload the contents from the tank, twice attempting to heat the TDI material. The shipment was rejected after the unsuccessful attempts at unloading, but the tank car was left in the unloading area until the explosion.
Samples of the original material and residue ejected during the incident revealed the presence of self-polymerized TDI, TDI monomer, and ureas. However, thermal analysis of the materials indicated that the polymerized TDI and TDI monomer had a thermal-runaway onset temperature that was much higher than possible with the steam heating system. A chemical hazard assessment revealed that the cause of the explosion was the runaway thermal decomposition of a contaminant mixed with the TDI waste. The TSDF operator relied on the waste generator for information regarding the waste composition and safe heating procedures. In this case, the waste generator supplied incomplete waste characterization data and inadequate instructions and warnings for heating and handling the TDI waste.
Fires at a municipal solid waste transfer facility
A rural municipal solid waste (MSW) facility was temporarily storing and sorting municipal and industrial waste prior to transfer to a landfill. During this time, two fires occurred in a three-day period. The first involved a trailer of waste awaiting transfer to the landfill, and the second involved a different trailer and a large portion of a building containing waste. After the first fire, the facility did not conduct a formal investigation, and relied on supposition and poor science to conclude that the incorrect waste stream was the cause. The incorrect conclusion was adopted by the facility and the real cause went unaddressed.
The facility operators commingled different materials, both in piles and in open trailers located in loading bays, and the commingled waste was periodically hauled to a municipal landfill. The facility did not accept RCRA hazardous waste or liquid wastes. The local regulations and the permit for the facility both acknowledged the reasonable possibility of hot loads, i.e., waste materials that undergo self-heating or are already smoldering.
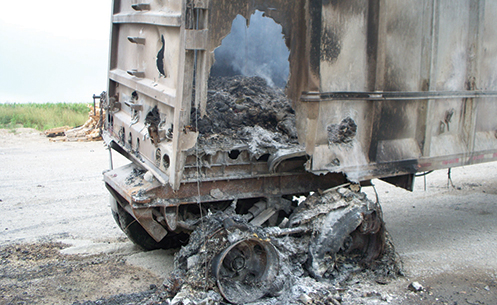
▲Figure 8. The fire originated in the rear of the trailer, possibly due to overheated brakes.
The first fire involved the contents and the rear of the trailer (Figure 8), which was located in a loading bay overnight. The trailer contained a mixture of bottom ash from a fluidized-bed coal combustor and other unknown MSW and construction debris. The facility moved the burning trailer away from the building, allowing it to extinguish on its own. Witness statements indicated that it burned for the next several days. The fire damage patterns on the trailer were consistent with a fire originating at the rear wheels, perhaps due to overheated brakes.
Three days after the first fire, a second fire was discovered in a different trailer loaded with similar waste. The trailer was held overnight in the same loading bay as the first trailer. This fire, however, destroyed the adjoining building.
An early investigation of the second fire concluded that the combustor bottom ash was incorrectly described as agricultural lime, a term that is commonly used to describe products that contain calcium carbonate (CaCO3), calcium hydroxide (Ca(OH)2), or calcium oxide (CaO). Instead, the site concluded that the waste was the equivalent of pure lime or quick lime (CaO), which they suspected underwent exothermic hydration, and the heat of that reaction started both fires. CaO should be classified as a RCRA hazardous waste.
The initial investigation concluded that the mischaracterization was the direct cause of the first fire. The engineering investigation conducted after the second fire analyzed that conclusion and also identified several potential causes of the fires, including an electrical fire, spontaneous combustion of MSW, and rekindling of the smoldering remains of the first fire (the trailer was close to the area where the first fire occurred). Chemical analysis of the combustor bottom ash and a review of the literature (16) indicated that the ash did not contain sufficient CaO to cause spontaneous combustion through hydration. Further, the waste was not a RCRA hazardous waste as defined by the federal hazardous-waste regulation 40 CFR 261.4(b).
The incorrect conclusion that the ash waste was CaO and that its exothermic hydration started the fires highlights the importance of precisely describing waste streams — even if the streams are not covered by the RCRA regulation — as well as the value of a systematic scientific investigation.
Additional thoughts
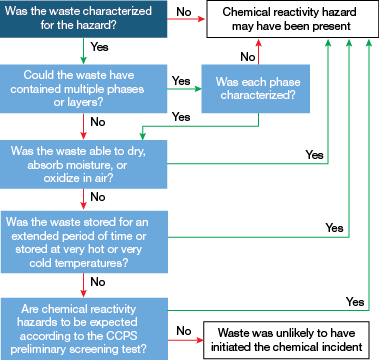
▲Figure 9. Use this decision tree to aid investigations of incidents that may involve hazardous waste.
As these incidents illustrate, investigating the cause of an accident involving hazardous waste is challenging. Figure 9 provides guidelines for investigating suspected waste streams and their role in initiating a fire or explosion.
Many of the accidents detailed here were caused by chemical reactions followed by a release of heat or pressure that caused a storage vessel to fail. While accurate waste characterization, adequate waste labeling, and suitable packaging materials are significant preventive safeguards against these types of failures, it may not always be possible to determine whether a waste stream is completely stable based on testing. Therefore, facilities may still need to consider the impact of a flammable vapor release or an exothermic and/or pressure-generating reaction within waste streams. Companies should consider thoughtful facility siting (e.g., using hazardous area classifications, adequate distance or barriers between waste streams), fire protection systems (i.e., flame and gas detectors), and limiting storage time prior to processing.
Oxidizers may also be involved in initiating or increasing the severity of incidents. Inerting atmospheres may be an effective and robust protective measure in many process operations; however, due to the potential for oxidizer ingress in misidentified waste, additional protections such as explosion vents may be required.
Training is also a vital protection against accidents, not only for TSDF personnel but also the waste generator’s personnel. Training should address the fundamental technical concepts associated with hazardous-waste materials, enabling waste coordinators to successfully implement RCRA and PSM requirements at their facilities.
Literature Cited
- Center for Chemical Process Safety, “Guidelines for Risk Based Process Safety,” CCPS, Wiley, Hoboken, NJ (2007).
- U.S. Environmental Protection Agency, “2015 Biennial Report Summary,” https://rcrainfo.epa.gov/rcrainfoweb/action/modules/br/summary/view (accessed Jan. 15, 2017).
- U.S. Environmental Protection Agency, “Waste Analysis at Facilities that Generate, Treat, Store, and Dispose of Hazardous Wastes — Final,” www.epa.gov/sites/production/files/2015-04/documents/tsdf-wap-guide-final.pdf (Apr. 2015).
- Johnson, R. W., et al., “Essential Practices for Managing Chemical Reactivity Hazards,” CCPS, Wiley, Hoboken, NJ (Mar. 2003).
- National Fire Protection Agency, “NFPA 921: Guide for Fire and Explosion Investigations,” NFPA, Quincy, MA (2017).
- Center for Chemical Process Safety, “Guidelines for Investigating Chemical Process Incidents, second edition,” CCPS, Wiley, Hoboken, NJ (2007).
- U.S. Chemical Safety Board, “Case Study: Fire and Community Evacuation in Apex, North Carolina,” www.csb.gov/assets/1/19/EQFinalReport.pdf, CSB, Washington, DC (Apr. 16, 2008).
- U.S. Chemical Safety Board, “Safety Advisory: Dangers of Unspent Aircraft Oxygen Generators,” www.csb.gov/assets/1/19/EQ_Safety_Advisory_Final.pdf, CSB, Washington, DC, (June 2007).
- Gray, B. F., and B. W. Halliburton, “The Thermal Decomposition of Hydrated Calcium Hypochlorite (UN 2880),” Fire Safety Journal,35 (3), pp. 223–239 (Oct. 2000).
- Gray, B. F., “Spontaneous Combustion and Self-Heating,” in “SFPE Handbook of Fire Protection Engineering,” DiNenno, P. J., et al., eds., Springer, New York, NY, pp. 211–228 (2002).
- Ogle, R. A, and A. R. Carpenter, “Toxic Gas Release Caused by the Thermal Decomposition of a Bulk Powder Blend Containing Sodium Dichloroisocyanurate,” Process Safety Progress,22 (2), pp. 75–82 (June 2003).
- Ogle, R. A., et al., “Missed Opportunities in Reactive Chemical Hazard Evaluations,” Process Safety Progress,25 (1), pp. 2–7 (Mar. 2006).
- National Transportation Safety Board, “Rupture of a Railroad Tank Car Containing Hazardous Waste Near Clymers, Indiana,” www.ntsb.gov/investigations/AccidentReports/Reports/HZM0101.pdf, NTSB, Washington, DC (Feb. 18, 1999).
- Ogle, R. A., et al., “Explosion of a Railcar Containing Toluene Diisocyanate Waste,” Process Safety Progress,23 (4), pp. 316–320 (Dec. 2004).
- Cox, B. L., et al., “Lessons Learned from Case Studies of Hazardous Waste/Chemical Reactivity Incidents,” Process Safety Progress,33 (4), pp. 395–398 (July 2014).
- Anthony, E. J., et al., “An Examination of the Exothermic Nature of Fluidized Bed Combustion (FBC) Residues,” Waste Management Journal,19 (4), pp. 293–305 (July 1999).
Copyright Permissions
Would you like to reuse content from CEP Magazine? It’s easy to request permission to reuse content. Simply click here to connect instantly to licensing services, where you can choose from a list of options regarding how you would like to reuse the desired content and complete the transaction.