A resurgence in the popularity of bourbon has inspired a more detailed study of the science involved in its production.
The bourbon industry has experienced a recent resurgence. Consider that the more than nine million barrels aging in Kentucky amounts to two barrels in inventory per state resident (1). While 95% of the world’s bourbon supply is produced in Kentucky (Figure 1), bourbon can be produced anywhere in the U.S.
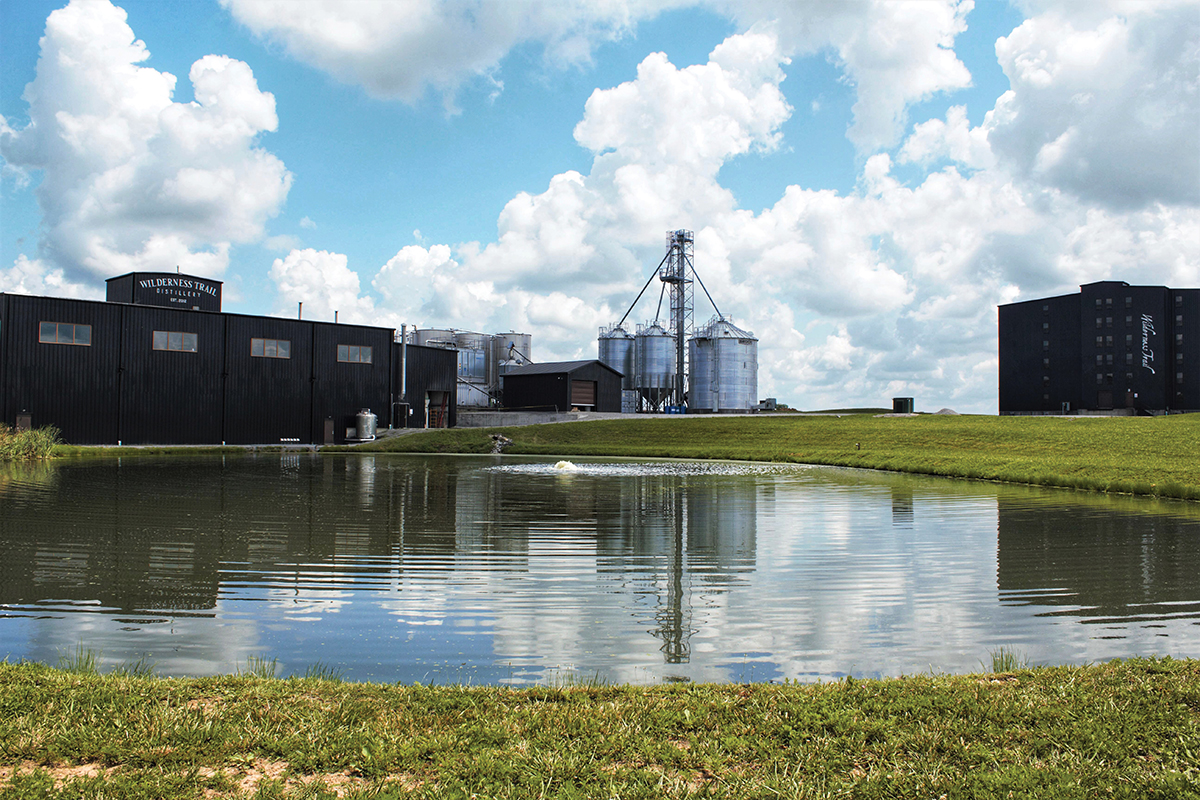
▲Figure 1. Wilderness Trail Distillery, a member of the famed Kentucky Bourbon Trail, is the fourteenth largest bourbon producer in the world with an annual capacity of more than 70,000 barrels per year.
Sections
Bourbon is a distilled spirit that begins as a mash bill (i.e., ratios of grains added to water) of at least 51% corn, which is fermented and distilled to no more than 160 proof — i.e., 80% alcohol by volume (ABV). Bourbon enters a new, charred-oak barrel at no more than 125 proof, and it is bottled above 80 proof.
While the definition of bourbon specifies that the spirit must be made from at least 51% corn, other grains such as rye, wheat, and malted barley are commonly used. The grains are ground and heated with water to create a mash, which is fermented with yeast that produce alcohol and other congeners (i.e., chemical components). This mixture is then distilled, barreled, and aged. During each step in this basic process, bourbon producers specify various technical details that affect the resulting product quality and attributes. This article explores the science and technology behind this process of turning a mash of corn and grains into a complex spirit.
Mashing
The first step in the production process is to grind the grains to produce the grist. The grist is transferred to a mash cooker (or mash tun), where it is mixed with water and heated in a process called mashing (Figure 2). Each type of grain is added individually at a temperature appropriate for the specific grain. The grains contain large amounts of starch, but the yeast used for fermentation are generally only able to metabolize glucose. Therefore, the mashing process helps to liberate glucose from the starch.
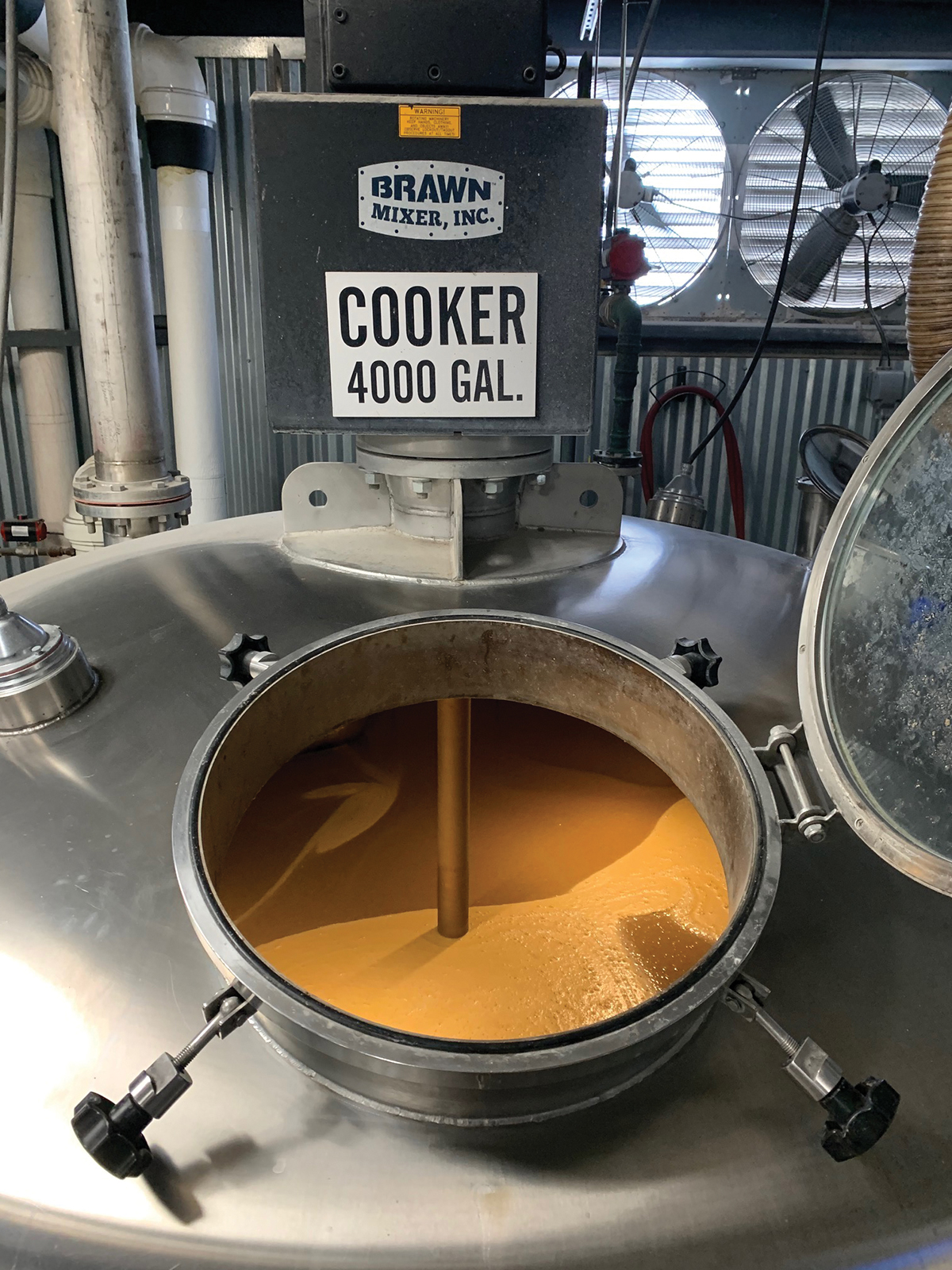
▲Figure 2. In the mash tun, the grains and water are heated with enzymes that break starches into fermentable sugars.
During mashing, several key transformations occur. As the water and milled grains are heated, water is forced into the grist in a process called gelatinization. Figure 3 presents microscope images of corn starch particles, which swell with increasing temperature and time. Initially, corn starch granules range from 7–20 µm, but over time as temperatures increase, the starches undergo liquefaction and the chains of starch begin to separate. Once the starch chains are liberated from the structure of the grist, saccharification occurs.
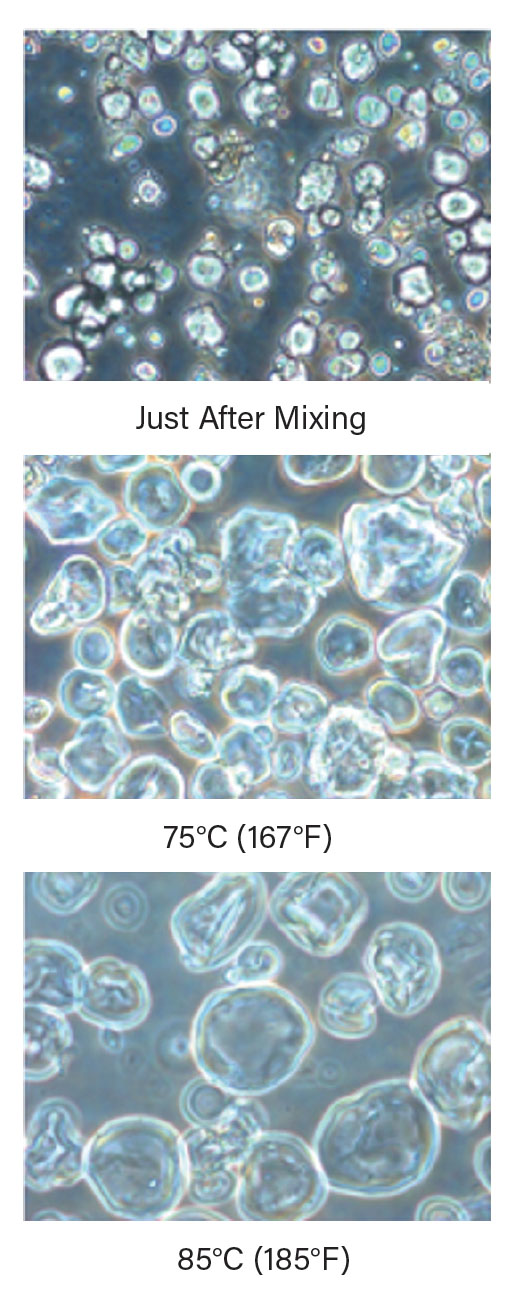
▲Figure 3. As the water and grains are heated, water is forced into the starch particles, causing them to swell and begin to break apart.
During saccharification, the chemical bonds of the polymerized starch are broken into simple sugars via an enzymatic reaction. Traditionally, the role of malt in a mash bill was to provide the necessary enzymes for this process. Malting causes the grains to begin the germination process. As part of germination, seeds produce enzymes to break down their starch stores to generate energy. By carefully allowing germination to start and then, just as it begins, stop, enzymes are generated, but the starch is not significantly consumed.
Today, commercially available enzymes are often used to increase the efficiency of the process. Specifically, a high-temperature alpha amylase is added during mashing to digest large starch polymers into smaller chains (i.e., dextrins) by cleaving the alpha-1,4 linkage in the starch. Alpha amylase is also used to reduce the viscosity of the mash, especially when the mash bill contains large amounts of rye. Glucoamylase enzymes are then added, further cleaving the dextrins into fermentable sugars, including maltose and glucose. The activity of these enzymes depends on the temperature and pH. Typical temperatures during the mashing process can be as low as 145–160°F for wheat, rye, and malted barley, or greater than 200°F for corn.
Fermentation
After the sugars have been liberated from the starch, the mixture is cooled and moved from the mash tun to a fermenter, where yeast is added (Figure 4). Some facilities that have a more continuous process recycle this cooling water to heat the next round of mash water.
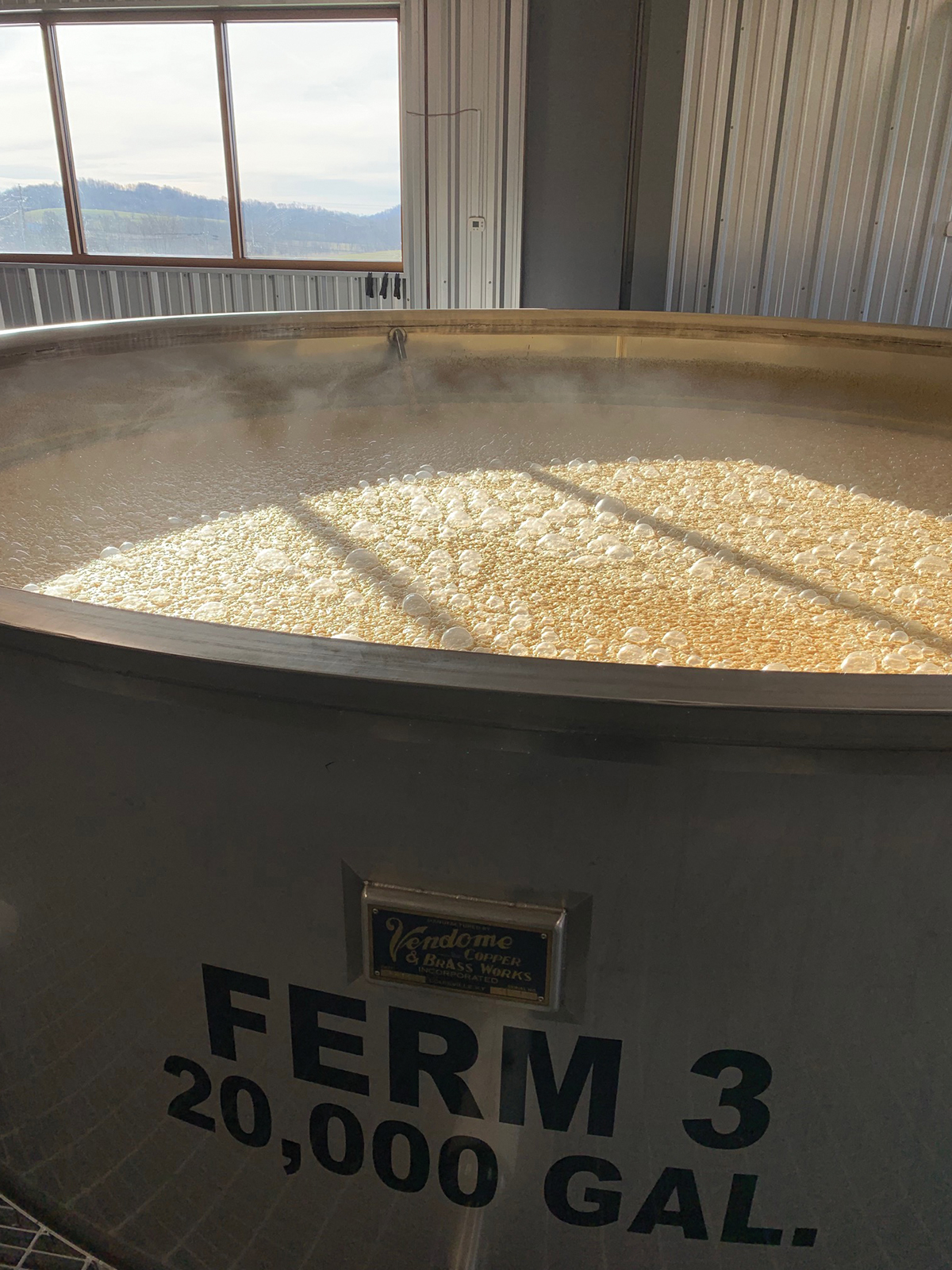
▲Figure 4. Traditionally, and often still today, fermentation vats are uncovered and open to the environment.
The distiller can make the mash in one of two ways: sweet or sour. In a sweet mash bourbon, only grains, water, and yeast are combined to make the mash. In a sour mash bourbon, part of the water is supplemented with stillage (i.e., setback), which is the liquid portion that remains from a previous distillation after the alcohol is removed. Because of the presence of acidic compounds (e.g., organic acids) formed throughout the fermentation process, setback is sour, hence the name of this process. Both methods can make great bourbons, but there is contention among distillers as to whether sweet or sour mash is best.
Although distiller’s yeast has the highest activity at temperatures above ambient (85–95°F), fermentation vats generally do not need to be warmed. Fermentation itself generates sufficient heat to reach optimum temperatures. Because yeast give off so much heat as they metabolize the sugars, the mash must actually be cooled to prevent it from getting too hot and affecting the health of the yeast. As yeast consume sugars, the primary products are ethanol and carbon dioxide. Generation of carbon dioxide in the fermentation process causes the mash to bubble and mix. Although the fermentation rooms are spacious, the large amount of carbon dioxide generated must be monitored and vented as a safety measure. Typically, the fermentation is active enough that distilleries do not use mechanical agitation.
Minor congeners are produced in the fermentation process, including higher alcohols and carboxylic acids. Esters are important flavor compounds that are produced directly by the yeast and any contaminating bacteria. Esters such as ethyl octanoate (apple), ethyl dodecanoate (floral), and isoamyl acetate (banana), as well as aldehydes and ketones all significantly impact flavor (Figure 5). If the boiling points of these chemical compounds are sufficiently close to the optimal distillation temperature for ethanol, they can be carried into the final product. Esters can also develop later in the aging process as alcohols condense with organic acids over time.
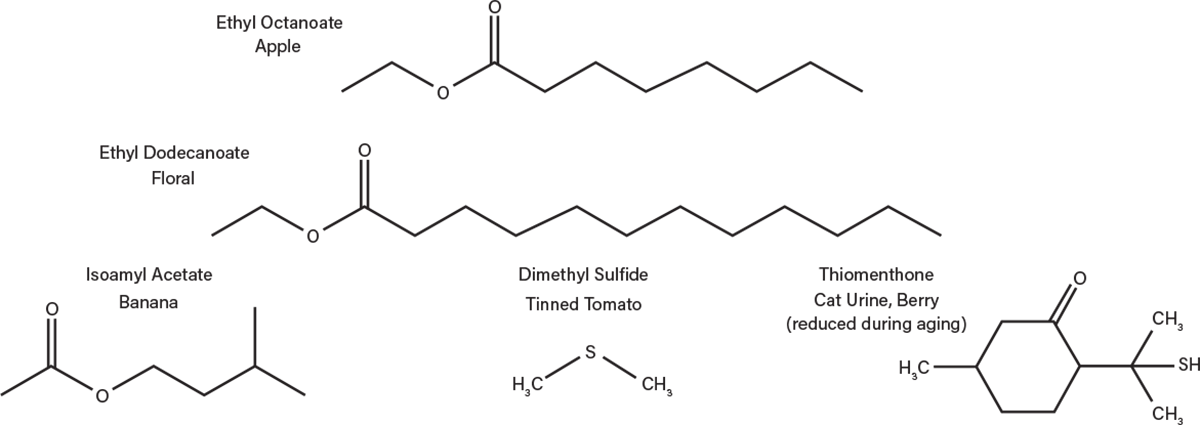
▲Figure 5. These are just a few of the flavor compounds that are generated during fermentation.
After a fermentation period of about 3–4 days, most of the sugars have been converted into alcohol, producing what is known as distiller’s beer (or wash). Unlike the beer we are familiar with, which contains residual sugars, distiller’s beer should be low in sugar, which will not be carried over in the distillate. In many modern distilleries, the sugar content, as well as other chemical markers of yeast and microbial growth, are monitored using high-performance liquid chromatography (HPLC) to ensure the health and productivity of the fermentation.
An important distinction between beer and bourbon production is the solids content of the fermentation broth. In beer production, solids are removed prior to fermentation, creating a clear wort. Whereas fermentations for bourbon are carried out “grain on,” meaning the solids are left in the fermenter. This is called a whole mash, which differentiates bourbon from Scotch and Irish whiskies, which are made by fermenting clear wort, as is the case with beer.
Distillation
Once fermentation is complete, the distiller’s beer is transferred to the still. The two main classes of stills include pot stills, which distill a single, relatively small batch, and column stills for continuous distillation. This article focuses on column stills, which are capable of higher throughput (Figure 6).
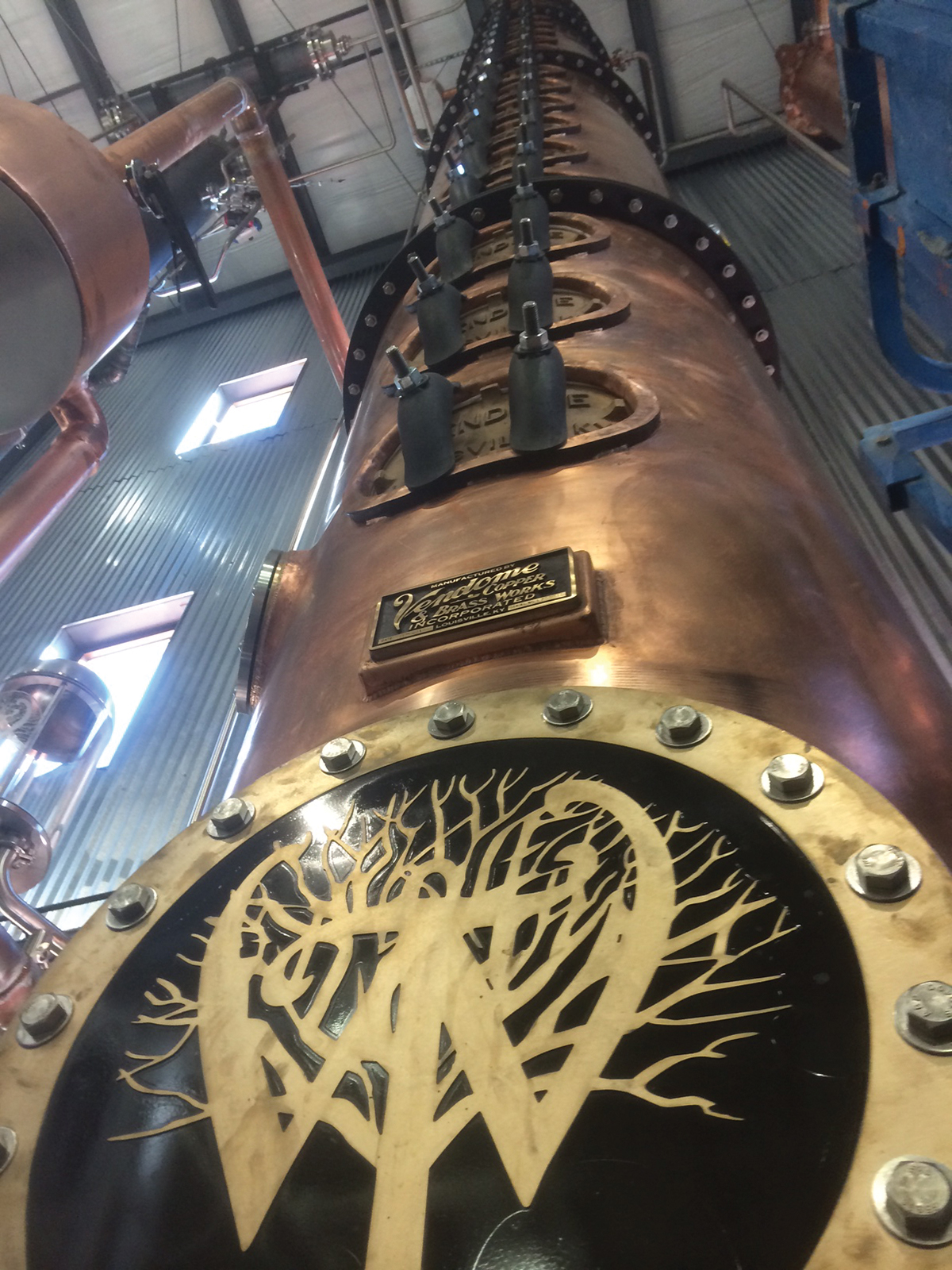
▲Figure 6. The stills used in bourbon production operate under the same principles as distillation columns used for separations throughout the chemical process industries (CPI). The stills are constructed of copper, which has antimicrobial properties, and reacts with sulfur to form copper sulfate, effectively removing sulfur from the distillate and improving flavor.
The distiller’s beer is added near the top of the column and steam rises from the bottom. As the distiller’s beer passes down the column across plates via downcomers, lower boiling point compounds, including ethanol, are stripped from the mixture and rise with the steam. As the temperature decreases up the column, the fraction of the distillate that contains the concentrated alcohol, usually at approximately 125 proof, is collected and condensed into “low wine.” The low wine then passes to a doubler (or thumper), which is a continuous pot still usually heated via steam coils. The distillate is collected at approximately 140 proof.
At the end of distillation, a large volume of stillage remains. The stillage contains remnants of grains, yeast cells, water, and other fermentation products, which are high in proteins and other nutrients. Stillage is commonly given away as feed for cattle and other livestock. In Kentucky, demand for this product is somewhat seasonal. In the winter, when pastures are fallow, demand is higher than in the summer when grass is readily available. A large distillery can generate millions of gallons of stillage per week. Wilderness Trail Distillery, for example, produces about 90,000 gal of stillage per day. The distillery recently became the first to employ innovative ultrafiltration technologies to reduce the moisture content of the stillage. This process significantly reduces the volume of stillage that needs to be transported, while at the same time reclaiming water, resulting in environmental and economic savings.
Barreling and aging
While the distillate can legally be distilled to 160 proof, it must enter a new, charred oak barrel at no greater than 125 proof. Therefore, the distillate is diluted prior to entering the barrel. For example, Wilderness Trail Distillery barrels most of its bourbon and rye whiskies at or below 110 proof. The clear, colorless distillate has only a portion of the flavor and none of the brownish amber color of a finished bourbon. The barrel and the aging process contribute significantly to the final flavor, mouthfeel, and color. The aging/maturation process typically takes at least four years, during which chemical extraction, chemical reactions, and evaporative loss occur.
Chemical extraction. The wood used to make the barrels is often seasoned (i.e., dried) for more than two years prior to construction of the barrels, which helps to break down larger wood components, making them more easily extractable. The staves are then toasted and charred to further break down components of the wood. Each distiller carefully selects the seasoning time and level of toasting and charring for their particular product.
Rickhouses or rackhouses (Figure 7), where the bourbon barrels are stored, are typically not climate controlled. Fluctuating seasonal temperatures cause the whiskey in the barrel to expand and contract, facilitating extraction. In the heat of summer, the bourbon expands and is physically pushed into the charred wood or even deeper into the uncharred wood of the barrel. In winter, the liquid contracts. These fluctuations are vital to changing the chemical composition of the bourbon.
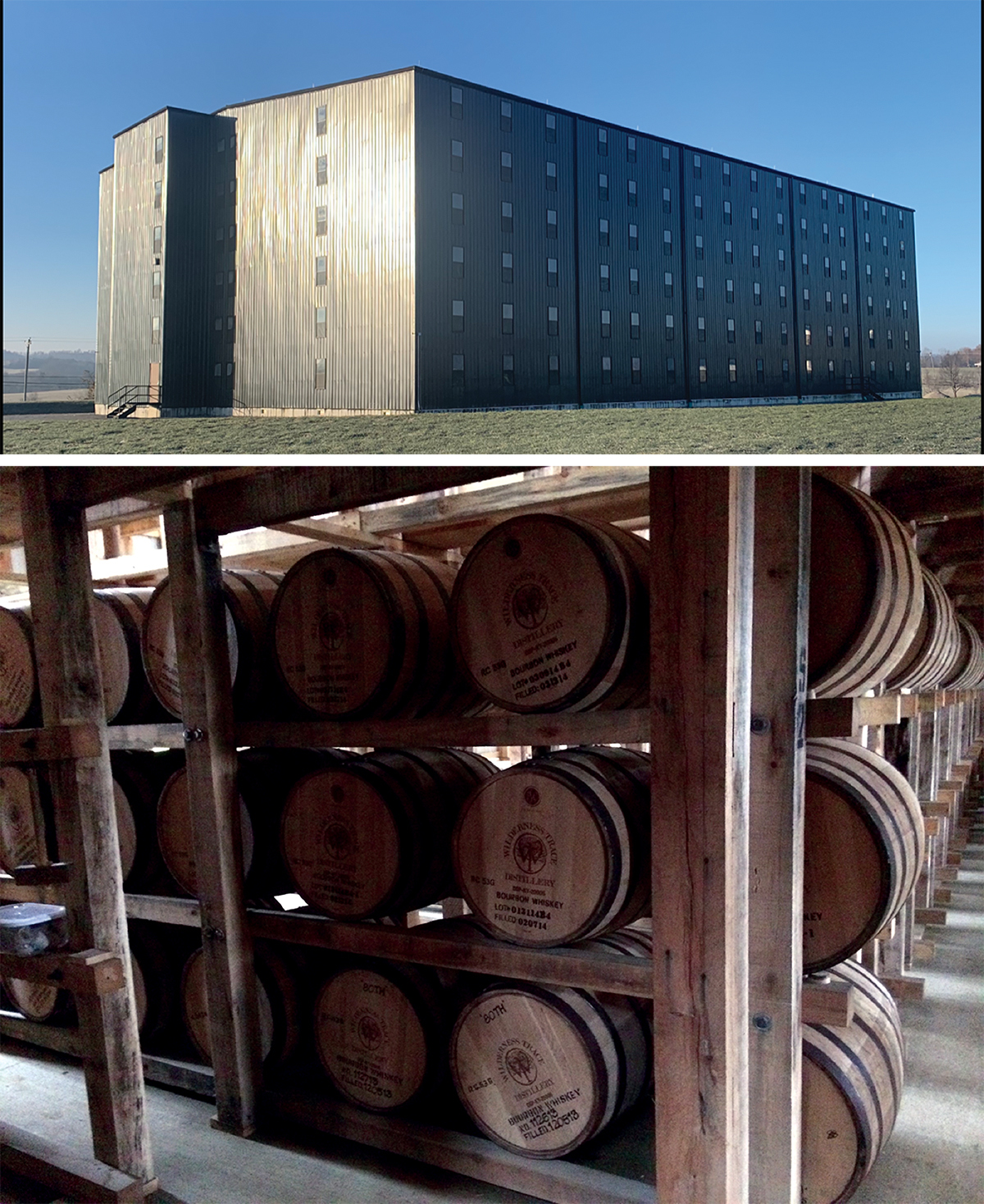
▲Figure 7. These photos show the outside and inside of a rickhouse that is not temperature controlled. The temperature variations cause the bourbon to expand and contract in the barrel.
Water in the spirit extracts large chemical compounds from the wood, many of which contribute flavor to the bourbon (Figure 8). For example, wood cellulose and hemicellulose are polysaccharides that can be broken down during the aging process. Sugars such as glucose, fructose, xylose, galactose, and arabinose have all been traced to contributions from barrels (2). Tannins, a term familiar to wine drinkers, are also extracted from the wood during aging. These compounds are extracted most quickly in the first year and are responsible for some of the astringency, bitterness, and mouthfeel of bourbon and wood barrel-aged wines. Wood lignin, present in wood layers below the char, is also degraded into a variety of compounds during aging, including vanillin, responsible for the flavor of vanilla.
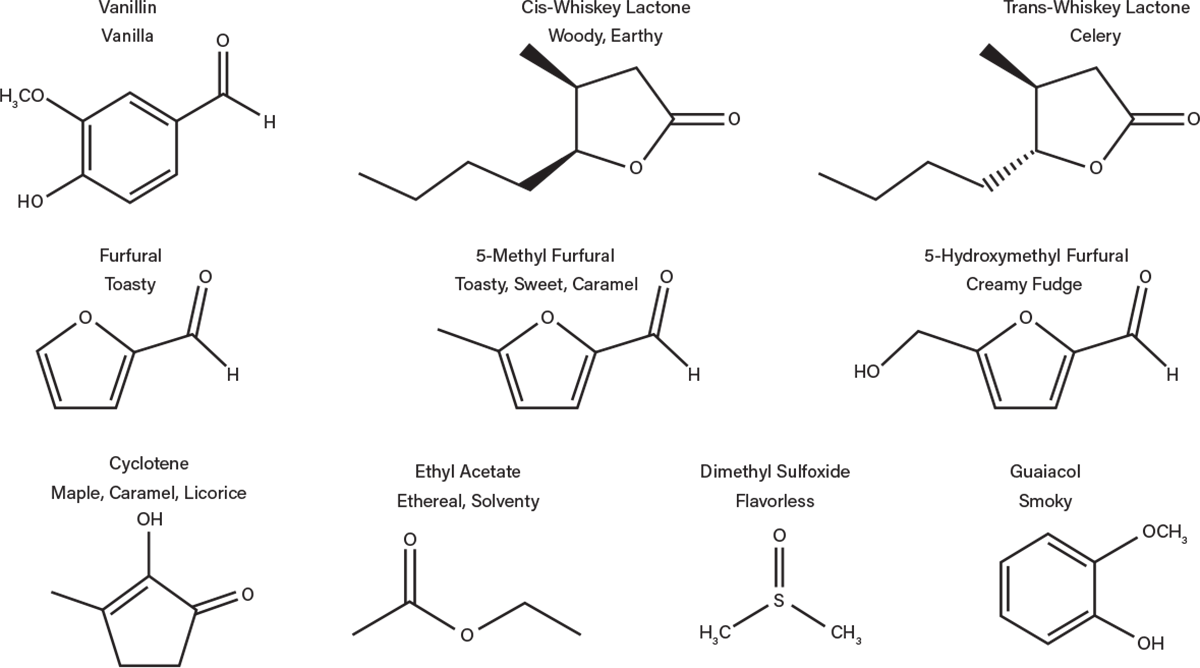
▲Figure 8. These are just a few of the compounds that are generated in the bourbon during aging in wood barrels.
Other compounds can be traced to both the wood and char layers. For example, oak lactones are volatile at charring temperatures, so they can be both driven off and formed during charring. This compound can occur in two enantiomeric forms. Interestingly, the cis form has a woody, earthy flavor and the trans form has a slight celery flavor. In a mixture of the two forms, as is present in bourbon, a slight coconut flavor can be noted (3). Other compounds that can be traced to a breakdown of components during charring are furfurals, imparting a caramel, toasted, and sweet flavor, and cyclotene, imparting maple, caramel, and licorice characteristics. All of these additions change the character of the final product.
Bourbon is required to be aged in a new barrel. In contrast, Scotch and Irish whiskies may be aged in used barrels — often used bourbon whiskey barrels. Many of the changes mentioned point to the necessity of the new barrel. As the compounds are removed from the barrel, they are not replaced. Thus, a second use of the barrels would result in lower concentrations of these components and a lower quality bourbon. For many compounds, the rate of extraction from the barrel slows over time.
Esterification. Esterification is an important set of chemical reactions that occur during aging. Organic acids are both extracted from the wood and present in the distillate. Given time, these organic acids can react with alcohols from the distillate to produce esters. For example, ethyl acetate increases over time from 160 ppm in the distillate to 500 ppm after six years in the barrel (4).
While esterification adds desirable flavors to bourbon over time, other reactions remove less-desirable compounds. Among the subtractive changes that occur during aging, an interesting example is dimethyl sulfide (DMS). DMS is derived during fermentation, likely from methionine in the grains. This compound has a tinned tomato flavor with a human detection threshold of 10–150 ppb. Because of its similarity to water, it is carried over into the distillate. Once thought to be lost purely through evaporation, isotopic analysis has shown that it is also oxidized to produce dimethyl sulfoxide (DMSO) (5). This conversion has been demonstrated to be related to interactions with the oak barrel (6). DMSO is essentially flavorless. Thus, chemical reactions over time remove the undesirable tomato flavor.
Another interesting sulfur compound that decreases during aging is thiomenthone. It is not uncommon for this congener to be present in the distillate above its flavor threshold. At concentrations higher than 1.3 ppm, it has an aroma similar to cat urine, while at low concentrations, 3 ppb, it has a berry flavor (5). Aging reduces the concentration of thiomenthone in bourbon, improving the overall flavor.
Evaporation. Some evaporative loss occurs during aging. Because water molecules are much smaller than alcohol molecules, water mostly escapes from the barrel, increasing the alcohol concentration. Evaporative loss depends on several factors — one of the most important is humidity. In Kentucky, it equates to around 2–3% loss per year.
Bottling and drinking
After aging, a distiller may batch multiple barrels together to maintain a consistent flavor profile (small-batch bourbon), or bottle from a single barrel (single-barrel bourbon). Bourbon must be bottled at greater than 80 proof. In many cases, water will be added to dilute the bourbon to the desired proof and achieve the preferred flavor profile. Bourbons with the bottled-in-bond designation are bottled at 100 proof. Some distillers and consumers prefer bourbon that is bottled at barrel or cask strength, generally greater than 110 proof.
Of course, the final step in the process is to pour a drink. The answer to the question of whether to enjoy the bourbon neat, with a splash of water, or on the rocks is a source of great debate. In actuality, each is backed by science. A splash of warm water increases the temperature, bringing out some of the more-volatile components into the headspace above the bourbon, thus concentrating them in the aroma. Similarly, the technique of holding the bourbon in your mouth, swallowing, and then breathing warms the bourbon, bringing the volatile components into the nasal cavity.
Back in the glass, water concentration and the addition of ice may have the unexpected consequence of concentrating aroma components in the headspace as well. One computational study looked at the partitioning of one component, guaiacol, in an ethanol-water mixture. At ethanol concentrations less than 45%, ethanol tends to concentrate at the liquid-air interface (7, 8). Additionally, guaiacol concentrates in ethanol micelles. Thus, in a slightly diluted bourbon, guaiacol would be expected to concentrate near the liquid-air interface and be more readily transmitted into the headspace above the bourbon. While this study was fairly limited, it is reasonable to expect that other semi-hydrophobic congeners would also partition into ethanol micelles.
Both experience and science tell us that the manner we sample our bourbon will affect the flavor, as do all the decisions made by the distiller in the production process. Which decisions lead to the best experience are up to those that imbibe this spirit of molecules.
Literature Cited
- Kentucky Distillers Association, “2 Barrels For Every Kentuckian — Distillers Top 9 Million Barrels For First Time in Modern Era,” https://kybourbon.com/2-barrels-for-every-kentuckian-distillers-top-9-million-barrels-for-first-time-in-modern-era (2019).
- Reazin, G. H., “Chemical Mechanisms of Whiskey Maturation,” American Journal of Enology and Viticulture, 32 (4), pp. 283–289 (1981).
- Koppenhoefer, B., et al., “Enantiomeric Odor Differences and Gas Chromatographic Properties of Flavors and Fragrances,” Perfumer & Flavorist, 19 (5), pp. 1–14 (1994).
- MacNamara, K., et al., “Flavour Components of Whiskey. II Ageing Changes in the High-Volatility Fraction,” South African Journal of Enology and Viticulture, 22 (2), pp. 75–81 (2001).
- Lee, K.-Y. M., et al., “Origins of Flavour in Whiskies and a Revised Flavour Wheel: A Review,” Journal of the Institute of Brewing, 107 (5), pp. 287–313 (2012).
- Masuda, M., and K. Nishimura, “Changes in Volatile Sulfur Compounds of Whisky During Aging,” Journal of Food Science, 47 (1), pp. 101–105 (1982).
- Karlsson, B. C. G., and R. Friedman, “Dilution of Whisky — The Molecular Perspective,” Scientific Reports, 7 (1), p. 6489 (2017).
- Karlsson, B. C. G., and R. Friedman, “Author Correction: Dilution of Whisky — The Molecular Perspective,” Scientific Reports, 8 (1), p. 16448 (2018).
Copyright Permissions
Would you like to reuse content from CEP Magazine? It’s easy to request permission to reuse content. Simply click here to connect instantly to licensing services, where you can choose from a list of options regarding how you would like to reuse the desired content and complete the transaction.