Sections
High-biocontainment labs carry out ongoing research on lethal diseases to prepare the U.S. for potential outbreaks.
Only a select few biology labs are equipped to study deadly pathogens such as Ebola, anthrax, and other dangerous diseases. Specialized high-biocontainment labs use state-of-the-art protective equipment and rigorous disinfection protocols to handle live pathogens capable of causing fatal illness. These high-biosafety labs study diseases that could cause catastrophic epidemics. In the past two years, many have devoted resources to studying SARS-CoV-2, the virus that causes COVID-19, alongside their ongoing research on emerging threats.
The Centers for Disease Control and Prevention (CDC) designate the biosafety level (BSL) of labs with a four-tiered scale (Table 1) (1). Labs at the lowest biosafety level, BSL-1, can study pathogens that do not usually cause disease. BSL-2 labs can study diseases with a moderate level of risk of illness. BSL-3 can study diseases that could cause death if inhaled, including respiratory viruses such as SARS-CoV-2. BSL-3 facilities use special ventilation systems and require personnel to wear masks and other personal protective equipment (PPE) to prevent airborne transmission.
Table 1. The Centers for Disease Control and Prevention (CDC) designate the biosafety level (BSL) of labs with a four-tiered scale. | |||
Safety Level | Description | Diseases Studied | Safety Considerations |
BSL-1 | Study of pathogens that do not usually cause disease | Non-infectious educational strains of Escherichia coli and diseases not known to affect humans such as certain plant and animal pathogens | Basic disinfection practices and personal protective equipment (PPE) such as gloves and lab coat |
BSL-2 | Study of diseases with a moderate level of risk of illness | Human immunodeficiency virus (HIV); Hepatitis A, B, and C; Salmonella; Zika | Biological safety cabinets (BSCs) that provide ventilated spaces to work with pathogens, doors that automatically close and lock, autoclave for decontaminating materials exposed to pathogens |
BSL-3 | Study of diseases that could cause death if inhaled | SARS-CoV-2; Middle East Respiratory Syndrome (MERS); Tuberculosis; West Nile virus; Yellow fever; Avian flu | Ducted air ventilation system with high-efficiency particulate absorbing (HEPA) filtration; PPE such as gowns/scrubs, masks and goggles/face shields, and replacing gloves whenever contaminated |
BSL-4 | Study of pathogens transmitted as aerosols that can cause deadly diseases for which there are no current cures | Ebola, Marburg, Crimean-Congo Hemorraghic Fever (CCHF), Lassa, and other hemorrhagic fevers; Smallpox (variola virus); Monkeypox; Eastern equine encephalitis (EEE); Bacillus anthracis (anthrax) | Airlocked entrances; changing clothes when entering; non-recirculating ventilation, airtight full-body PPE suit connected to external air supply; showering when exiting |
Finally, only BSL-4 labs can study pathogens transmitted as aerosols — tiny droplets that can stay in the air for hours — and that can cause deadly diseases for which there are no current cures. These include Ebola, Marburg, variola virus (smallpox), anthrax, monkeypox, and other diseases with high mortality rates. The entrances to BSL-4 labs have airlocks, and workers must wear full-body suits connected to external air supply. BSL-4 labs are a crucial part of preparing the U.S. for potential outbreaks and creating vaccines, drugs, and diagnostic tools for use in other countries where outbreaks have occurred.
“BSL-4 labs have been built to be part of the response to national crises in the infectious disease sector,” says Heinz Feldmann, the chief of the virology lab at Rocky Mountain Laboratories. “Nature is full of surprises. But that doesn’t mean we shouldn’t be prepared.”
The COVID-19 pandemic has underscored the need for preparedness against emerging diseases. This article explores how BSL-4 labs in the U.S. are seeking to identify potential pandemic threats, invent treatments to stop lethal pathogens from spreading out of control, and communicate their findings to the public to increase awareness and understanding of infectious disease research.
From a glovebox to a full-scale lab
Jean Patterson, a professor at Texas Biomedical Research Institute (Texas Biomed), began working with BSL-4 pathogens with only a glovebox facility — a small sealed-off space with thick gloves inserted into the box. After the 2001 anthrax attacks, in which letters carrying live anthrax bacteria killed five people, federal funding to study bioterrorism threats helped Texas Biomed expand its BSL-4 facility to a larger lab space.
Patterson’s research group primarily studies hemorrhagic fevers, which are viral diseases that cause death by uncontrolled bleeding (hemorrhaging). These include Ebola and Marburg, related viruses that belong to the filovirus family (Figure 1). Both have an average mortality rate of about 50% in infected people (2, 3). “The military has always had a concern that [Marburg and Ebola] could have been used as bioterrorism agents,” Patterson says.
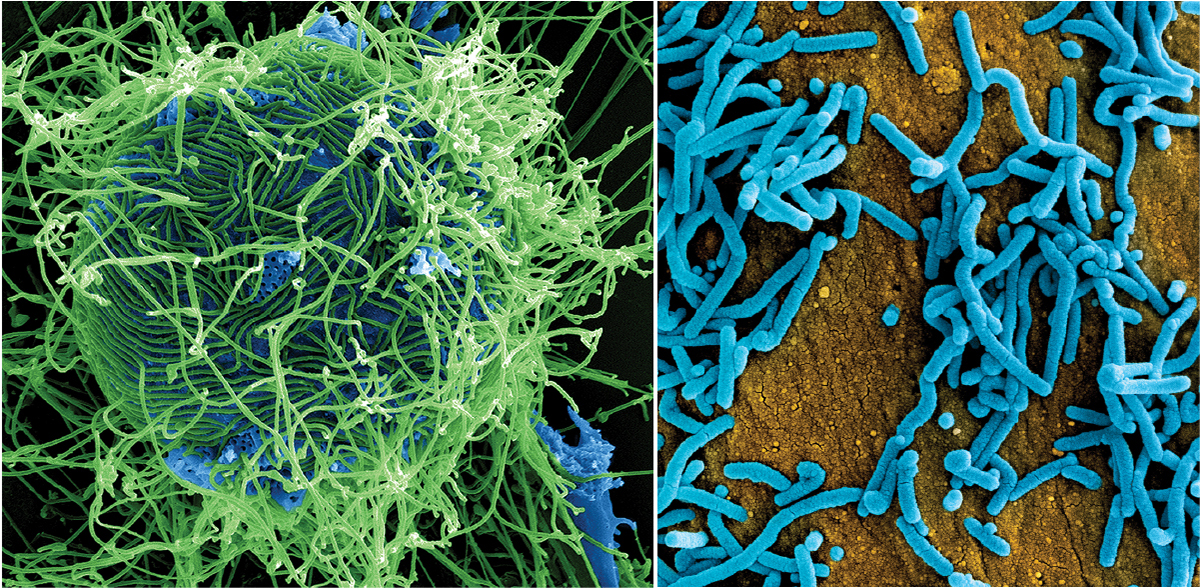
▲Figure 1. Ebola and Marburg viruses cause hemorrhagic fevers. At left is a colorized scanning electron micrograph of filamentous Ebola virus particles (green). At right, a colorized scanning electron micrograph of Marburg virus particles (blue) is shown. Images courtesy of the National Institute of Allergy and Infectious Diseases (NIAID).
Making vaccines and drugs for deadly diseases like Ebola can be challenging. The lethality of filoviruses and the short duration of many outbreaks means that researchers often use animal models of disease to test new treatments. The U.S. Food and Drug Administration (FDA) has a policy called the Animal Rule, stating that when it is infeasible or unethical to conduct a traditional human clinical trial, researchers can study a new treatment in two animal models of the disease, typically one non-primate mammal and one primate. Animal models must develop a disease similar enough to the human form of the disease to provide a good indication of how humans would respond to the treatment (4). If a drug or vaccine shows success in animal models, then the U.S. government can begin to stockpile it.
Patterson and colleagues established a way to study Ebola and Marburg infections in common marmosets, a small New World monkey commonly used in animal trials in biomedical research. The researchers confirmed that marmosets exposed to filoviruses contract a disease very similar to humans, making them a good model for testing new treatments (5).
The marmoset model allowed Patterson and colleagues to develop three different vaccines for Ebola and two vaccines for Lassa virus, which is found in western Africa, transmitted by rodents, and can cause hemorrhaging, deafness, and organ failure (6). These vaccines could be deployed in future outbreaks if the need arises. Now, Patterson is testing a Zika vaccine in pregnant marmosets to determine whether it protects both the mother and fetus from Zika.
Patterson says researchers are now focused on designing treatments that are universal, meaning that they target broadly shared traits in multiple different species or strains of viruses. For example, an antiviral could target a trait common to viruses as different as influenza, Dengue fever, and SARS-CoV-2, she says.
During the COVID-19 pandemic, Patterson’s lab has collaborated with other researchers on SARS-CoV-2 research, investigating how the lungs of primates respond to severe COVID-19 infection to gain a better understanding of how the virus causes serious illness (7).
Patterson and her colleagues are also committed to outreach efforts to raise awareness of their research. While there is mistrust of infectious disease research among some members of the public, Patterson says she has seen success in local outreach efforts, particularly within San Antonio, TX, where Texas Biomed is located. At educational events, Patterson and her colleagues describe the rigor of BSL-4 safety protocols and the importance of preparing for threats like Ebola.
“Building good community relations is absolutely critical,” she says. “We went to a lot of community events — Rotary Club and Optimist Club meetings — and told everyone what we were doing and why we were doing it. People seemed quite comfortable with it.”
New ways of attacking viruses
At one of the newest BSL-4 labs in the U.S., the National Emerging Infectious Diseases Laboratory (NEIDL) at Boston Univ., associate professor John Connor is developing antiviral drugs for the national stockpile to defend against bioterrorism threats. To identify highly effective antivirals, Connor’s lab studies how viruses hijack the processes of human cells to reproduce. Learning how pathogens interact with their human host cells could reveal ways to disrupt the viral lifecycle.
Some experiments involve interrupting part of an infected cell’s metabolism and seeing how the virus reacts. Recently, Connor and colleagues found that Ebola needs organic molecules called polyamines to express its genes. Reducing the levels of polyamines in the cell with a chemical called difluoromethylornithine blocked gene expression in the virus (8). “This essentially opens up an entirely new way of trying to attack severe viral infections through controlling cell metabolism, which we hadn’t previously considered,” he says.
During the pandemic, Connor has contributed to research on how human lung cells respond to SARS-CoV-2 infection, which could illuminate new targets for antiviral drugs to reduce the virus’s lifespan (9).
Connor’s lab is carrying out similar work in studying how Lassa virus interacts with human cells to cause disease, which is not fully understood. Scott Seitz, a postdoctoral researcher in Connor’s lab, is undergoing training for the BSL-4 lab to work with Lassa virus.
Every time Seitz enters the BSL-4 lab, he has to wear a full-body suit, often called a “spacesuit,” that connects to external air supplies delivered from hoses hanging from the ceiling (Figure 2). A 400-ft2 lab area may have four separate air supply hoses, meaning Seitz often disconnects from one hose and reconnects to another as he moves around the room. “All of your movement is very space-like,” Seitz says. “You’re moving very slowly.”
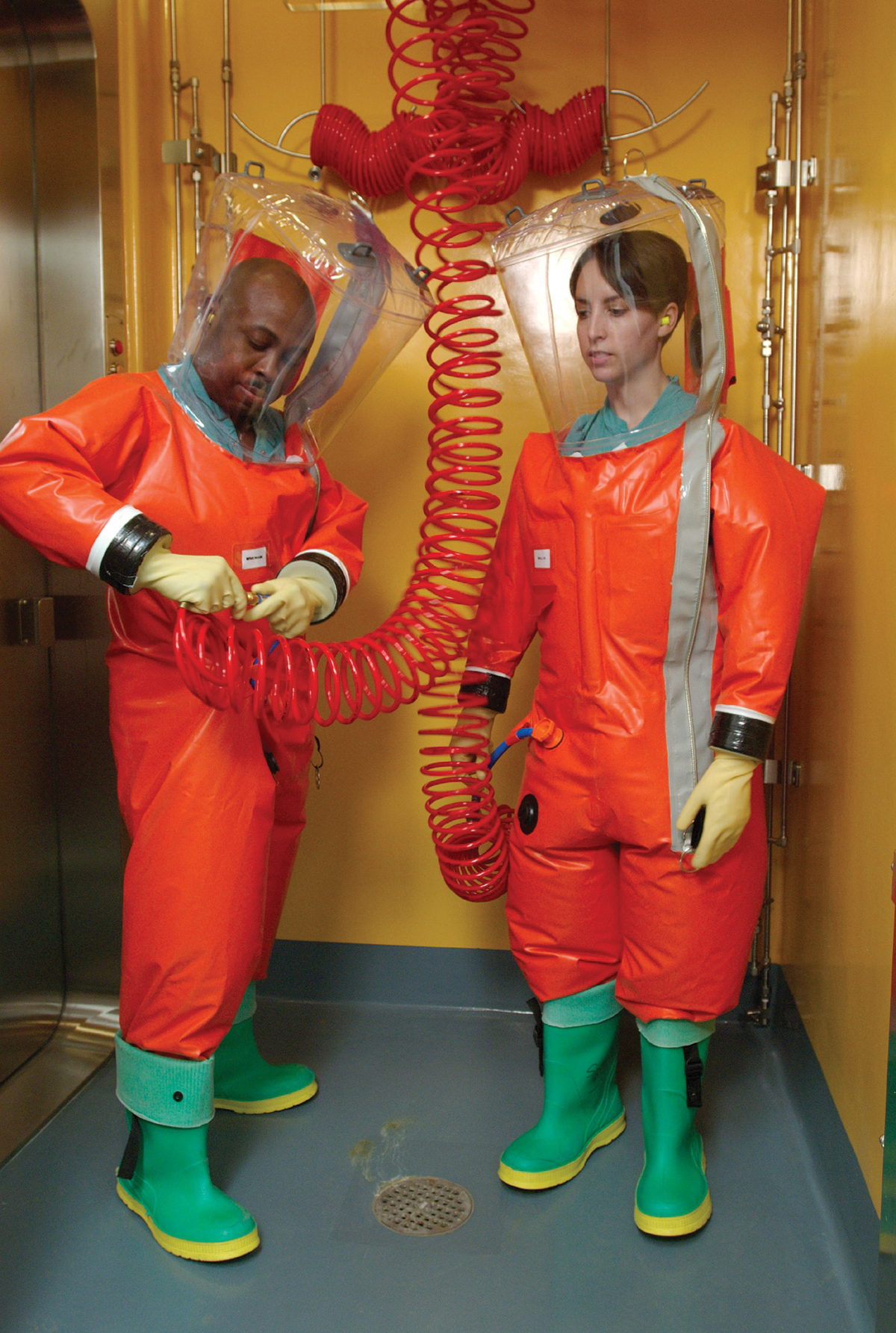
▲Figure 2. To work in a BSL-4 lab, researchers are required to wear a full-body suit that is connected to an external air supply. Image courtesy of James Gathany and the Centers for Disease Control (CDC).
In addition to practicing normal lab techniques in the protective suit, trainees also learn to handle medical emergencies like fainting or heart attacks in the lab. Seitz says it’s crucial to treat every object in the lab as if it were a needle that could cause a puncture and disease exposure.
The precautions limit the pace of activities in a BSL-4 lab. “The joke is that if it takes an hour at BSL-2, it will take two hours at BSL-3, and four hours at BSL-4,” Seitz says. Researchers carefully plan out their BSL-4 experiments to use time efficiently.
Family and friends often ask Seitz about his work. He sees their questions as chances to serve the public and increase understanding of BSL-4 research. He has given presentations to middle school and high school classes, as well as people who manage animals used for research. He enjoys the questions he receives in those presentations, because they force him to consider why someone thinks differently than he does. Understanding the origins of people’s concerns can help when communicating the value of infectious disease research and vaccine/antiviral development, he says.
“Trying to understand why someone sees things the way they do is a good starting place versus [focusing on] why you already think they’re wrong,” Seitz says. “That’s never worked for me in a teaching environment.”
While many people in North America likely do not worry about hemorrhagic fevers, the COVID-19 pandemic has shown the importance of preparing for the unlikely event of an outbreak of a disease like Ebola, Connor says. “The goal of doing research now on these viruses is to have defenses in place to make certain that they never become a pandemic,” he says.
Old diseases in new places
Predicting which pathogen will cause the next major crisis has been challenging in the past, says Feldmann. “If you go back to the middle of the 90s, everyone expected avian influenza to become the next big epidemic or pandemic — that never happened,” Feldmann says.
It’s most likely that the next major disease emergency will be caused by a virus with lower lethality than Ebola but a high rate of infection like SARS-CoV-2, Feldmann says. Though less likely, hemorrhagic fever outbreaks would cause great harm if they ever did occur, making it worth studying them and preparing for an outbreak.
Feldmann’s lab is studying a disease called Crimean-Congo Hemorrhagic Fever (CCHF), a virus carried by a type of tick called Ixodid or “hard” ticks. The disease is found in southern and eastern Europe, parts of Asia and the Indian subcontinent, and throughout Africa (Figure 3). CCHF causes hemorrhaging; estimates of the mortality rate from past outbreaks range from 9–50% of infected patients (10).
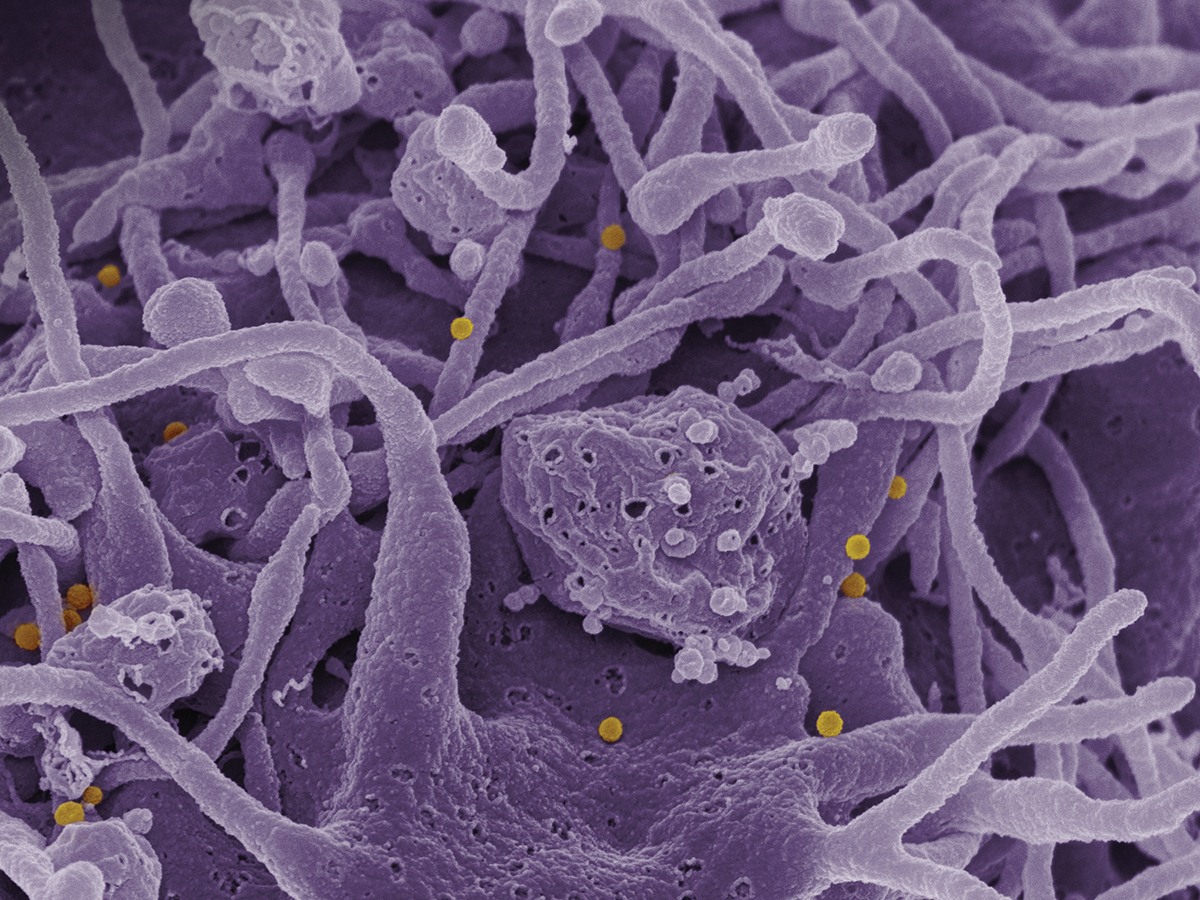
▲Figure 3. In this scanning electron micrograph image, Crimean-Congo hemorrhagic fever (CCHF) viral particles (yellow) bud from the surface of cultured epithelial cells from a patient. Image courtesy of the National Institute of Allergy and Infectious Diseases (NIAID).
Tick bites can transmit CCHF, but people also become exposed through contact with livestock animals with the virus in their blood. For that reason, South Africa quarantined ostriches before processing them at slaughterhouses to contain a CCHF outbreak in 1996 (11).
Climate change is allowing the tick hosts of CCHF to increase their range further into the Northern Hemisphere. Feldmann says the spread of hard tick species into other parts of the world raises the possibility of CCHF outbreaks in new areas.
“We’re not just worried about the diseases that may appear one day that we don’t know yet. We’re also worried about diseases that we know quite well occurring in areas of the world that have not seen them before,” Feldmann says. There is also concern over whether tick species in North America could carry and transmit CCHF if they are exposed.
Knowledge of how CCHF interacts with host cells is lacking. Feldmann and colleagues have developed animal models of CCHF so that researchers can test vaccines, drugs, and diagnostic tools using the live virus (12). The researchers are working on vaccines for both high-risk people, such as agricultural and meat-processing workers, and the livestock themselves.
Feldmann’s lab has contributed to research on SARS-CoV-2. Feldmann and colleagues demonstrated that hydroxychloroquine is not an effective treatment for COVID-19 in animal models of the disease (13), and helped test an experimental viral vector vaccine delivered as a nasal spray (14).
While Feldmann’s lab studies infectious diseases, they are also informing the local community of Hamilton, MT, about their research. Scientists at Rocky Mountain Laboratories host open houses, public lectures, and presentations for local healthcare providers so that they understand the procedures should someone become infected.
“The best way is always full transparency,” Feldmann says, noting that BSL-4 research is not classified or secret. Some conspiracy theorists still speculate about mysterious activities in high-containment labs, but Feldmann notes that anyone can readily find abundant public information about BSL-4 research online.
Always ready
The ongoing work on dangerous pathogens lets BSL-4 labs provide the capacity for a fast and effective response to an infectious disease emergency, rapidly shifting their focus to the pathogen causing the crisis.
“We’re preparing and training our staff … so that in the case of an emergency, we can immediately mobilize and switch to the pathogen that’s causing a public health incident,” Feldmann says. “I think this is vital — to be operational all the time, to be ready if something happens. I think the public should know that the capacity is here, particularly in the U.S., and that this can be called upon any time it’s needed. I think that’s quite reassuring.”
Literature Cited
- Centers for Disease Control and Prevention and the National Institutes of Health, “Biosafety in Microbiological and Biomedical Laboratories, 6th Edition,” www.cdc.gov/labs/pdf/SF__19_308133-A_BMBL6_00-BOOK-WEB-final-3.pdf (2020).
- World Health Organization, “Ebola Virus Disease,” Geneva, Switzerland, https://www.who.int/news-room/fact-sheets/detail/ebola-virus-disease (2021).
- World Health Organization, “Marburg Virus Disease,” Geneva, Switzerland, https://www.who.int/news-room/fact-sheets/detail/marburg-virus-disease (2021).
- U.S. Food and Drug Administration, “Animal Rule Approvals,” www.fda.gov/drugs/nda-and-bla-approvals/animal-rule-approvals (2021).
- Carrion, R., Jr., et al., “A Small Nonhuman Primate Model for Filovirus-Induced Disease,” Virology, 420 (2), pp. 117–124 (2011).
- Centers for Disease Control and Prevention, “Lassa Fever,” Atlanta, GA, www.cdc.gov/vhf/lassa (2019).
- Singh, D. K., et al., “Responses to Acute Infection with SARS-CoV-2 in the Lungs of Rhesus Macaques, Baboons and Marmosets,” Nature Microbiology, 6, pp. 73–86 (2021).
- Olsen, M. E., et al., “Differential Mechanisms for the Involvement of Polyamines and Hypusinated eIF5A in Ebola Virus Gene Expression,” Journal of Virology,92 (20), pp. e01260-18 (2018).
- Hekman, R. M., et al., “Actionable Cytopathogenic Host Responses of Human Alveolar Type 2 Cells to SARS-CoV-2,” Molecular Cell, 80 (6), pp. 1104–1122 (2020).
- Centers for Disease Control and Prevention, “Crimean-Congo Hemorrhagic Fever,” Atlanta, GA, www.cdc.gov/vhf/crimean-congo/index.html (2013).
- Swanepoel, R., et al., “Experimental Infection of Ostriches with Crimean-Congo Haemorrhagic Fever Virus,” Epidemiology & Infection, 121 (2), pp. 427–432 (1998).
- Hawman, D. W., et al., “Immunocompetent Mouse Model for Crimean-Congo Hemorrhagic Fever Virus,” eLife, 10, pp. e63906 (2021).
- Rosenke, K., et al., “Hydroxychloroquine Prophylaxis and Treatment is Ineffective in Macaque and Hamster SARS-CoV-2 Disease Models,” JCI Insight, 5 (23) pp. e143174 (2020).
- Hassan, A. O., et al., “A Single Intranasal Dose of Chimpanzee Adenovirus-Vectored Vaccine Protects Against SARS-CoV-2 Infection in Rhesus Macaques,” Cell Reports Medicine, 2 (4), pp. 100230 (2021).
Copyright Permissions
Would you like to reuse content from CEP Magazine? It’s easy to request permission to reuse content. Simply click here to connect instantly to licensing services, where you can choose from a list of options regarding how you would like to reuse the desired content and complete the transaction.