Despite plastic’s many benefits, the ever-increasing mass of unmanaged plastic waste is causing significant damage to the global ecosystem. A few key regulations, as well as recycling technologies, are helping to curb the threat.
Plastics are everywhere. In fact, it is nearly impossible to go about your day without coming into contact with plastic. From household appliances to electronics to the synthetic fibers in your clothes, plastics make modern life possible. In fact, life as we currently live it is not possible without these amazing polymers.
Produced in huge quantities, plastics have transformed our world in countless ways. Plastic packaging has reduced transportation costs by improving fuel economies as manufactured goods are moved around the globe. Plastics have revolutionized food packaging, allowing food to stay fresh longer and dramatically reducing food loss. Better building insulation has increased energy savings. In the world of medicine, disposable plastic instruments and hermetically sealed plastic packaging have greatly improved sanitation. From a consumer standpoint, plastics have increased convenience and reduced product costs. Goods made from traditional materials like wood, cotton, wool, stone, leather, metal, and glass have all lost market share to competing products made from plastic.
Our appetite for plastic shows no sign of relenting. Plastics have revolutionized nearly every industrial sector since the end of World War II. They also play a role in nearly every commercial sector. For example, plastics are integral to our food products — from polyethylene cling film to the blow-molded plastic bottles that hold beverages. Although packaging is the largest single user of plastic, accounting for nearly 30% of U.S. municipal solid waste according to the U.S. Environmental Protection Agency (EPA), no industry is untouched. Plastics are in our homes — in our carpets, on our nonstick cookware, and in our furniture. Plastic is in vehicles — in seating, paneling, instrument enclosures, and coverings. Vinyl window cladding, insulation on electrical wires, synthetic rubber in tires, medical devices, and personal protective equipment (PPE) are critical products that rely on plastic. All of these and thousands of other products are made of plastic.
Plastic is stable, durable, chemically inert, easily formable, lightweight, and, most importantly, inexpensive. However, it can persist in the ecosystem for hundreds or even thousands of years. Decades of mismanagement have resulted in discarded plastics — often post-consumer, single-use items — encroaching on every ecosystem on the planet. This accumulation of plastic waste has reached a crisis point, and immediate action is needed to stem the tide of plastic entering the global environment.
This article discusses some of the key issues facing the chemical process industries (CPI) with regard to waste plastic. Although plastic is not a hazardous waste in the traditional sense, it presents an immediate threat to the environment. Plastic can be recycled, but as explained here, it usually is not.
This article gives an overview of the challenges associated with recycling post-consumer-use plastics. It summarizes some of the key pieces of international authority designed to curb the threat presented by unmanaged plastic waste, and concludes with a discussion of two technologies that may improve our ability to recycle plastic.
The global accumulation of plastic waste
Despite plastic’s many benefits, the ever-increasing mass of unmanaged plastic waste is causing significant damage to global ecosystems. In fact, almost every piece of plastic ever made is still in existence. Plastic waste has been largely unmanaged due to its inert nature. Environmental policy addressing waste has historically prioritized restrictions to mitigate acute hazards from toxic or otherwise hazardous wastes, such as ignitable, reactive, or corrosive wastes.
The inert nature of plastic leaves it largely unregulated under U.S. federal laws, such as the Resource Conservation and Recovery Act, which addresses hazardous waste, and the Comprehensive Environmental Response, Compensation and Liability Act (CERCLA or Superfund), which addresses releases of hazardous substances. Due to plastic’s inert nature, most plastic is not covered under the Toxic Substances Control Act (TSCA) or internationally by the Registration, Evaluation, Authorisation and Restriction of Chemicals (REACh) regulation.
The perpetual existence of plastic has not, until recently, been recognized as a hazard in its own right. Thus, it is regulated merely as solid waste and litter by local authorities. Without comprehensive plastic waste regulation, emphasis has been placed on recycling plastic solely for its value upon recovery. However, although plastic certainly can be recycled, today only a tiny fraction of it actually is.
If not recycled, plastic waste needs to be properly managed. In industrial economies with well-developed infrastructure, plastic, along with other solid waste, typically ends up in landfills. However, plastic waste can escape from even well-developed solid waste management systems as litter. Underdeveloped countries often lack such waste-management infrastructure. If underdeveloped countries collect plastic waste at all, the waste is still likely to end up in an open dump, where winds or rain can sweep it away. And virtually anywhere in the world, if someone has to walk a long distance to find a proper disposal location, they are likely to drop the waste onto the street or in a ditch.
Once waste plastic enters the ecosystem, it persists for decades or even centuries. Some estimates predict that by the year 2050, humans will have produced 26 billion tons of plastic waste. Much of this plastic waste ends up in the ocean. It has become clear that the traditional model of produce ➝ distribute ➝ consume ➝ discard is unsustainable. This paradigm is in need of immediate and rapid change.
Recognition of risk and changes in global policy
Since the 1970s, global conventions have historically addressed pollution and litter to prevent ocean dumping and preserve the marine environment. Such conventions include the 1973 International Convention on the Prevention of Pollution from Ships (MARPOL) and the 1982 United Nations (UN) Convention on the Law of the Sea (UNCLOS).
More recently, the plastic waste crisis has spurred global partnerships specifically focused on mitigating plastic debris, such as the 2012 Global Partnership on Marine Litter, which the U.S. joined through its Trash-Free Waters Program implemented through federal Clean Water Act authority.
Other efforts followed, such as:
- In 2017, the U.S. Dept. of Energy launched the REMADE (Reducing EMbodied-energy And Decreasing Emissions) Institute.
- In 2018, the European Union (EU) released its European Strategy for Plastics in a Circular Economy.
- The American Chemistry Council (ACC) announced its adoption of plastics economy goals for plastic packaging in 2018.
- The World Economic Forum mobilized a new partnership, the Global Plastic Action Partnership (GPGP), which encourages collaborations between governments and stakeholders to redesign the global take-make-dispose economy into a circular economy.
- The Ellen McArthur Foundation launched its New Plastics Economy initiative, which details a vision for a circular economy for plastics and unites more than 1,000 organizations.
- In 2019, the Alliance to End Plastic Waste pledged $1.5 billion over five years to end plastic waste in the environment.
However, more immediately impactful has been China’s 2018 National Sword Policy, which bans the import of most plastic waste material. This action has had a ripple effect around the world that has not been resolved. Before this policy was enacted, shipping containers that left China full of manufactured consumer goods were returned filled with recyclables, including plastic. In China, the materials were sorted, separated, and recycled into new products. The National Sword Policy abruptly put an end to this cycle.
Also critical are the 2019 Amendments to the Basel Convention on the Control of Transboundary Movements of Hazardous Wastes and Their Disposal (often simply called the Basel Convention). The 2019 Amendments, for the first time, list plastic waste as conditionally hazardous (1). Effective January 2021, these amendments will trigger restrictions and prohibitions on plastic waste shipments for the 53 country parties to the Convention, including the 37 members of the Organisation for Economic Co-operation and Development (OECD) (1). (Generally, OECD members have high-income economies and are regarded as developed countries.) Parties can no longer import plastic waste listed as hazardous waste from nonparties, or ship listed hazardous waste to and from nonparties without bilateral or regional agreements equivalent to the Basel Amendments. These restrictions will require reconfiguration of all high-volume plastic waste generators’ trade routes (1).
Although the U.S. is an OECD member, it has never ratified the Basel Convention and is not a Basel Party. In the case of the 2019 Basel Amendments, the U.S. objected to the OECD’s normal process of automatically adopting the most recent Basel Amendments (1). As a result, the U.S. stands alone, creating confusion regarding the legality of its own plastic waste exports. However, the other OECD members are expected to comply with Basel Amendments.
In this context, the risks of plastic waste in the environment have become better defined. The National Geographic series Plastic or Planet, as well as widely disseminated research regarding sources, fate, and transport of microplastics, have given us a better understanding of the extent of the problem. Plastic is everywhere — from the depths of the Mariana Trench to the snows of Antarctica. It exists in our water, in our air as fine particulates, in our food, and now even in us. Scientists are just now defining dose-response parameters to assess the toxicological impacts from microplastic exposure (2).
The global community has responded with its own wave of plastic manufacturing, use, and waste prohibitions. A 2018 UN review revealed that 127 out of 192 countries have adopted legislation to regulate plastic bags, and 63 countries mandate extended producer responsibility for single-use plastics. In 2019, the UN launched its Global Chemicals Outlook, calling for full disclosure, sound recycling, waste management, and sustainable product design. In 2020, the EU adopted its Circular Economy Action Plan to reduce waste by supporting circularity, creating secondary raw material markets, and addressing waste exports, building on 2018 and 2019 actions that prohibited single-use plastics, set consumption targets, and promoted waste regeneration systems. Canada and Mexico have adopted their own plans for single-use plastic, as well as waste prohibitions and circular economy approaches.
Within the global response, recycling is merely part of the solution, implemented along with manufacturing restrictions that support circular economy concepts.
Challenges to a circular plastics economy
Circular plastics economy approaches are intended to ensure that plastic never becomes waste or enters the ecosystem. For example, one approach might be to redesign products and materials to ensure their recovery and reuse, which will in turn reduce environmental impacts. Such circular economy approaches are exemplified by the EU Action Plan, the Global Plastic Action Partnership, and the Ellen McArthur Foundation, among others.
For example, in the New Plastics Economy initiative, the Ellen McArthur Foundation establishes the circular plastics economy with three goals:
- eliminate all problematic and unnecessary plastic items
- innovate to ensure that the plastics we do need are reusable, recyclable, or compostable
- circulate all the plastic items we use to keep them in the economy and out of the environment.
However, achieving these goals, which are common to most circular economy approaches, is complicated by the many formulations of plastic available on the market. Although most types of plastics are generally recyclable, in practice, this is not a simple proposition. In the U.S., thousands of formulations of plastic can be found on the market, which makes sorting and separating especially challenging. Additionally, not all formulations are easily recycled, which adds another level of complexity.
Most consumers in the U.S. are likely familiar with the seven numerical classifications stamped on consumer plastic products:
- Type 1: polyethylene terephthalate (PET), e.g., plastic beverage bottles
- Type 2: high-density polyethylene (HDPE), e.g., 1-gal milk jugs
- Type 3: polyvinyl chloride (PVC), e.g., pipes used in plumbing, vinyl tubing, and wire insulation
- Type 4: low-density polyethylene (LDPE), often found in plastic sheets or packaging (e.g., bread bags)
- Type 5: polypropylene (PP), often found in bottle caps, packaging, and plastic furniture
- Type 6: polystyrene (PS), e.g., drinking straws, beverage lids, and Styrofoam
- Type 7: other nonrecyclable plastics and all thermoset plastics (e.g., acrylics, nylons, polycarbonates, acrylonitrile butadiene styrene [ABS], and polylactic acid).
Significantly, these classifications are only a small part of the story. There are literally thousands of different formulations of plastic, each with a unique, often proprietary, blend of additives.
In most plastic products, the basic polymer is incorporated into a formulary with different additives, which are used to improve the performance, functionality, and aging properties of the base polymer. Additives can be divided into four main categories (3):
- functional additives (e.g., stabilizers, antistatic agents, flame retardants, plasticizers, lubricants, slip agents, curing agents, foaming agents, catalyst deactivators, nucleators, biocides, etc.)
- colorants (e.g., pigments, soluble azocolorants, etc.)
- fillers (e.g., mica, talc, kaolin, clay, calcium carbonate, barium sulfate)
- reinforcements (e.g., glass fibers, carbon fibers).
The most common additives in polymeric packaging materials are plasticizers, flame retardants, antioxidants, acid scavengers, light and heat stabilizers, lubricants, pigments, antistatic agents, slip compounds, and thermal stabilizers. Each of these plays a distinct role in delivering and/or enhancing the functional properties of a plastic product.
For instance, catalyst deactivators neutralize any remaining catalyst residues, nucleators increase resin clarity and reduce processing time, and pigments impart color. Antistatic agents permit the discharge of static electricity from film, and flame retardants allow the use of polypropylene in electronics, construction, and transportation applications. Antiblock and slip agents are commonly used in films, to prevent them from sticking together or to metal surfaces.
Additives complicate recycling. In order to recycle a post-use plastic back into virgin resin, the additives must be identified, separated, and properly disposed of. This is a daunting task, given the wide array of plastic formulations. This challenge, however, does provide potential opportunities for the CPI.
Opportunities for the chemical industry
The polymer chains that make up plastic contain significant quantities of embodied energy. This energy is a resource that so far has been largely untapped. Recycling rates in the U.S. remain low; incineration is the most mature method of reducing the amount of plastic waste, but it is typically more expensive and environmentally hazardous than landfilling.
Although mixed plastics can be incinerated for energy, this process often creates carcinogenic products and environmental pollutants. Thus, only 12% of waste is incinerated in the U.S. In addition, incineration does not take full advantage of the potential that these polymers hold.
Figure 1 shows the temperature and pressure ranges for some of the available technologies for processing plastic waste. Generally, the reaction mechanism of gasification, pyrolysis, and hydrothermal processing (HTP) is thermolysis (i.e., applying heat to depolymerize plastics). In particular, pyrolysis of plastics into plastic-derived fuel oil (PDFO) is relatively mature technology that recovers some of the embodied energy in plastic. However, it, too, misses out on the potential to reuse post-consumer plastic waste as a feedstock for producing new plastics.
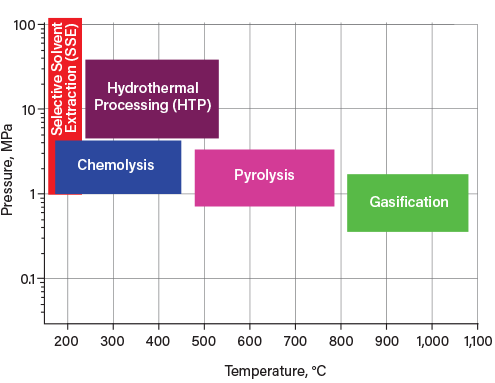
▲Figure 1. Opportunities abound for technological advancement in the field of chemical recycling. Here, the temperature and pressure ranges of different advanced plastic recycling methods are shown.
Unlike thermolysis, which uses heat, chemolysis uses chemicals to depolymerize polymers. Notably, chemolysis only works for condensation polymers such as PET and polyurethane. Condensation polymers are equilibrium materials, where addition of the condensation product (e.g., ethylene glycol) and heat reverses the polymerization. In other words, the principle behind chemolysis is to initiate a reverse reaction of the condensation reactions. Thus, chemolysis cannot depolymerize additive polymers like polyethylene (PE) and PP.
Chemolysis reactions include aminolysis, glycolysis, and methanolysis. Notably, selective solvent extraction (SSE) and chemolysis work well for sorted plastics and condensation polymers, respectively. However, they cannot be used to treat mixed plastic.
Opportunities abound for technological advancement in the field of chemical recycling. In particular, SSE and HTP show significant promise and are explored further in the following sections.
Selective solvent extraction
Solubility parameters are numerical estimates of the degree of interaction between compounds. Typically, Hansen solubility parameters (HSP) are used to predict the solubility of a targeted polymer in a solvent through the pair’s polar, hydrogen bonding, and dispersion parameters. Based on the HSP theory, an engineer can design a solvent-based process to extract targeted polymers by the dissolution-reprecipitation technique. In this technique, a polymer is dissolved in one solvent and heated to a designated temperature. The mixture is then cooled and put into a non-solvent — i.e., a solvent that cannot dissolve the targeted polymer but is miscible with the first solvent. The polymer can then be reprecipitated from the non-solvent and analyzed. In previous studies, solvents have been used to chemically recycle the polymers from plastic packaging (Figure 2) (4–6).
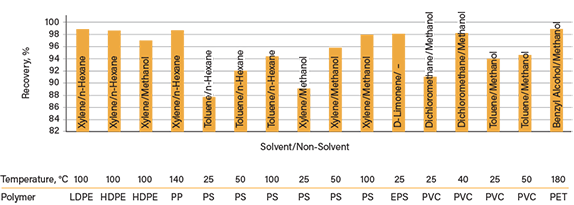
▲Figure 2. Through the process of selective solvent extraction (SSE), different solvents and non-solvent pairs were used to dissolve and reprecipitate polymers from plastic packaging. Recoveries up to 98% were possible. Source: Adapted from Refs. 4–6.
As Figure 2 depicts, xylene and toluene appear to be good solvents, while hexane and methanol work well as anti-solvents to recover the majority of the polymers (HDPE, LDPE, and PS) with high yields. On the other hand, dichloromethane (i.e., methylene chloride) and benzyl alcohol work well to dissolve plastics like PVC and PET. In addition, it is worth noting that Figure 2 also indicates that the dissolution temperature is important for achieving a high polymer recovery yield. However, one thing missing from the literature is the structure-activity relationship. For instance, how the crystallinity, additive compositions, and molecular weight may affect the dissolution/reprecipitation technique is unknown.
Despite some reports of success in the literature, knowledge gaps remain:
- The ability of SSE to tolerate impurities is unknown. Most of the research used relatively clean plastic for tests.
- The relationship between the solvent extraction conditions and the polymer dissolution behavior is unclear. For instance, it is unclear how the dissolution temperature, dissolution time, and solvent-to-polymer ratio may affect the solubility of a polymer. Accurate prediction models for polymer dissolution are limited.
- The solvents used in previous studies were relatively toxic (see Figure 2, for example). There is a critical need to explore safer solvent systems.
Hydrothermal processing
HTP is a thermochemical depolymerization process that takes place in an enclosed reactor, where water can serve as a solvent, catalyst, or reactant. HTP usually requires moderate temperatures (280–450°C) and pressures (7–30 MPa) (7). As the reaction condition approaches the critical point of water, its properties, such as dielectric constant, ionic strength, density, and heat/mass transport coefficients, change rapidly. In particular, the rapid change of density reflects changes at the molecular level such as solvation power, molecular diffusivity, and viscosity (7). These significant changes enable the subcritical or supercritical water to bring about fast, selective, and efficient reactions to convert organic wastes to crude oil (8–10).
Similar to pyrolysis, HTP favors polyolefins, but it can handle higher amounts of non-polyolefins plastics, including PVC and PET. Few studies have focused on using supercritical water to liquefy polyolefins into oil or gas products in the past two decades or so (11, 12). Only recently, the use of supercritical water for converting polyolefins into useful products has been explored. Chen et al. used HTP to convert PP into gasoline-like fuels and naphtha (13), while Jin et al. used a similar strategy to valorize PE waste into fuels, naphtha, and wax (14). Very recently, Seshasayee and Savage used HTP to convert PET, PP, PS, and polycarbonate into fuels (15).
Halogens are a serious challenge for thermochemical conversions such as pyrolysis. Halogens, once released from plastics, dissolve in water and form hazardous acidic compounds (e.g., HCl). Compounds generated by halogens can corrode reactors and pollute the final products. To abate this risk, researchers can add neutralizing agents or a co-solvent under HTP. Recently, HTP, along with supercritical fluids or neutralizing agents, have been used to remove plastic additives such as halogens from municipal solid waste (16, 17). However, no systematic study exists to guide the selection of appropriate neutralizing agents or co-solvents. Fundamental knowledge about how to engineer, design, and predict the solubility parameters of various neutralizing agents or co-solvents under HTP is therefore needed.
Several other knowledge gaps remain for industrial-scale deployment of HTP, such as:
- The interactions of certain plastics and other components under HTP are unknown. This could become an issue because mixed plastics usually contain other materials in a composite structure.
- The impact of impurities on HTP is unclear. In particular, halogenic compounds or brominated flame retardants may react with hydrocarbons and produce halogenated alkanes or aromatics.
Recently, researchers have considered applying HTP to per- and polyfluoroalkyl substances (PFAS) (18, 19). According to the EPA, there is evidence that exposure to PFAS can lead to adverse human health effects. Therefore, this is an area of considerable interest due to developing regulations regarding PFAS.
Despite challenges, HTP presents a tremendous opportunity for upcycling mixed plastic waste.
Waste plastic and the developing world
In many ways, plastic has improved life in the developing world. Plastics have improved the storage life of food products, revolutionized building construction, and transformed medical care. However, managing the waste generated by single-use plastics has been a major challenge.
Research by Jambeck et al. and Joshi et al. concludes that the vast majority of the mismanagement of plastic waste occurs in developing countries (Figure 3), though plastic waste still leaks into the environment in developed countries as litter (20, 21). Some of the specific challenges in developing countries include lack of infrastructure for trash collection, lack of strong governmental institutions to manage and enforce management policies, low emphasis on ecosystem protection, and low emphasis on human health vs. economic opportunity. Waste management approaches that work in OECD nations are unlikely to succeed in developing regions.
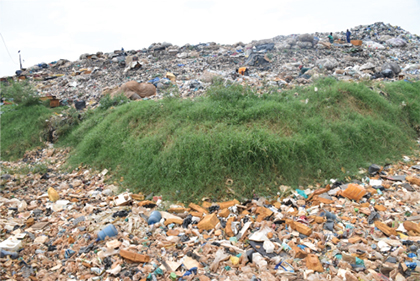
▲Figure 3. Plastic waste accumulates at the Kiteezi Landfill in Kampala, Uganda. Photo courtesy of J. Seay.
Effective waste reduction and management require the planning and development of infrastructure. Mass production of single-use plastic goods and goods that shed plastic during use, such as tires, fabrics, and coatings, must be reconsidered, given the risk of leakage into the environment. Consumers need choices to avoid generating plastic waste, and for the plastic waste that remains, they need convenient, local disposal options. Locally disposed waste must be collected at regular intervals. Public or private enterprise is needed to manage the collection and disposal of municipal solid waste in adequately designed and operated landfills.
Regions with infrastructure limitations face significant difficulties in managing waste. The current global plastic crisis was caused in part by the history of OECD nations sending mixed or contaminated waste to developing countries that lack the infrastructure to properly handle that waste. Global plastic regulation, including China’s National Sword Policy, the 2019 amendments to the Basel Convention, and the wave of circular economy mandates, necessitates that OECD nations, including the U.S., must chart a more disciplined course in manufacturing plastic goods and dealing with the accumulation of their plastic waste.
Potential pathways forward
Implementing a circular economy for plastics will require significant changes to the status quo. Despite their problems, plastics provide significant benefits to global sustainability, particularly in transportation, and there are no alternatives ready for immediate deployment at global scales. Therefore, plastics are going to be in our lives for the foreseeable future. Clearly, we as a global society want to continue to enjoy the benefits that plastics bring while we stop the unchecked flow of waste into the ecosystem. The circular economy approaches charted by the EU Action Plan, the Global Plastic Action Partnership, and the Ellen McArthur Foundation, among others, outline a similar roadmap for the chemical industry: elimination, innovation, and circularity.
Elimination. From a CPI perspective, elimination will entail a dramatic reduction in the number of plastic formulations. As discussed earlier, additives are a key reason recycling is so difficult; reducing their number can reduce the scope of the challenge. Of course, reducing the number of additives used will require compromise by consumers and producers alike. Finding leadership on this issue will be a vital first step.
Innovation. Technological breakthroughs in chemical recycling have the potential to be transformative. The science is still developing in this field and there are many knowledge gaps, but chemical recycling is a field of study that can and should be developed and explored.
Circularity. Design for circularity is an emerging field that will require manufacturers to rethink how products are designed. The point at which the consumer is finished using the product cannot be the end of the product’s life. Reuse, recycle, and remanufacturing principles must be applied during the product design phase. Single-use must be the exception rather than the rule.
For practical reasons in our intertwined global economy, this roadmap will likely become more or less compulsory for all plastic producers and products.
The road ahead
In short, a combination of international policy and consumer demand for change will necessarily slow the accumulation of mismanaged plastic waste. Globally, change is moving forward. However, the U.S. is still holding to its status quo and has failed to adopt national legislation to comprehensively address waste plastics, even though this is an area of critical importance.
There are challenges, of course, but also opportunities for innovation. The next 50 years of plastics manufacture and use must look significantly different from the past 50 if we are to avoid a global ecological disaster.
Literature Cited
- Ternes, M.E., “Plastics: Global Outlook for Multinational Environmental Lawyers,” Natural Resources & Environment, 35 (Oct. 5, 2020).
- Koelmans, A., et al., “Risk of Plastic Debris: Unravelling Fact, Opinion, Perception and Belief,” Environmental Science & Technology, 51 (20), pp. 11513–11519 (Oct. 2017).
- Hahladakis, J. N., et al., “An Overview of Chemical Additives Present in Plastics: Migration, Release, Fate and Environmental Impact During Their Use, Disposal and Recycling,” Journal of Hazardous Materials, 344, pp. 179–199 (Feb. 2018).
- Achilias, D. S., et al., “Recycling of Polymers from Plastic Packaging Materials Using the Dissolution-Reprecipitation Technique,” Polymer Bulletin, 63 (3), pp. 449–465 (2009).
- Achilias, D. S., et al., “Chemical Recycling of Plastic Wastes Made from Polyethylene (LDPE and HDPE) and Polypropylene (PP),” Journal of Hazardous Materials, 149 (3), pp. 536–542 (2007).
- Reimonn, G., et al., “Review of Microplastic Pollution in the Environment and Emerging Recycling Solutions,” Journal of Renewable Materials, 7 (12), pp. 1251–1268 (2019).
- Peterson, A. A., et al., “Thermochemical Biofuel Production In Hydrothermal Media: A Review of Sub- and Supercritical Water Technologies,” Energy & Environmental Science, 1 (2008).
- Kraft, S., and F. Vogel, “Estimation of Binary Diffusion Coefficients in Supercritical Water: Mini Review,” Industrial & Engineering Chemistry Research, 56 (16), pp. 4847–4855 (Mar. 2017).
- Savage, P. E., “A Perspective on Catalysis in Sub- and Supercritical Water,” The Journal of Supercritical Fluids, 47 (3), pp. 407–414 (Jan. 2009).
- Wang, Y., and F.-S. Zhang, “Degradation of Brominated Flame Retardant in Computer Housing Plastic by Supercritical Fluids,” Journal of Hazardous Materials, 205-206, pp. 156–163 (Feb. 2012).
- Moriya, T., and H. Enomoto, “Characteristics of Polyethylene Cracking in Supercritical Water Compared to Thermal Cracking,” Polymer Degradation and Stability, 65 (3), pp. 373–386 (Sept. 1999).
- Watanabe, M., et al., “Polyethylene Conversion in Supercritical Water,” The Journal of Supercritical Fluids, 13 (1–3), pp. 247–252 (June 1998).
- Chen, W.-T., et al., “Use of Supercritical Water for the Liquefaction of Polypropylene into Oil,” ACS Sustainable Chemistry & Engineering, 7 (4), pp. 3749–3758 (Jan. 2019).
- Jin, K., et al., “Conversion of Polyethylene Waste Into Clean Fuels and Waxes Via Hydrothermal Processing (HTP),” Fuel, 273, #117726 (Aug. 2020).
- Seshasayee, M. S., and P. E. Savage, “Oil from Plastic Via Hydrothermal Liquefaction: Production And Characterization,” Applied Energy, 278, p. 115673 (Nov. 2020).
- Chen, W.-T., et al., “A Perspective on Hydrothermal Processing of Sewage Sludge,” Current Opinion in Environmental Science & Health, 14, pp. 63–73 (Apr. 2020).
- Zhang, C. C., and F.-S. Zhang, “Enhanced Dehalogenation and Coupled Recovery of Complex Electronic Display Housing Plastics by Sub/Supercritical CO2,” Journal of Hazardous Materials, 382, #121140 (Jan. 15, 2020).
- Yu, J., et al., “Fate of Per- and Polyfluoroalkyl Substances (PFAS) during Hydrothermal Liquefaction of Municipal Wastewater Treatment Sludge,” Environmental Science: Water Research & Technology, 6 (5), pp. 1388–1399 (2020).
- Zhang, W., et al., “Destruction of Perfluoroalkyl Acids Accumulated in Typha latifolia through Hydrothermal Liquefaction,” ACS Sustainable Chemistry & Engineering, 8 (25), pp. 9257–9262 (2020).
- Jambeck, J. R., et al., “Plastic Waste Inputs from Land into the Ocean,” Science, 347 (6223), pp. 768–771 (2015).
- Joshi, C., et al., “A Perspective on a Locally Managed Decentralized Circular Economy for Waste Plastic in Developing Countries,” Environmental Progress & Sustainable Energy, 38 (1), pp. 3–11 (2018).
Copyright Permissions
Would you like to reuse content from CEP Magazine? It’s easy to request permission to reuse content. Simply click here to connect instantly to licensing services, where you can choose from a list of options regarding how you would like to reuse the desired content and complete the transaction.