Chemical and physical disinfection of feedwater to a reverse osmosis (RO) membrane helps to prevent fouling and maintain efficient operation.
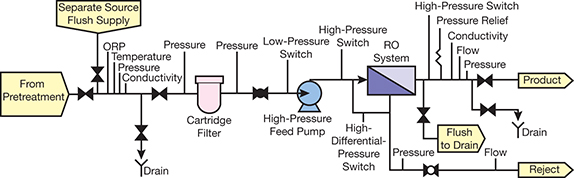
▲Figure 1. This is a typical reverse osmosis (RO) schematic. Biocides or other biofouling preventive measures are added to the feedwater during pretreatment.
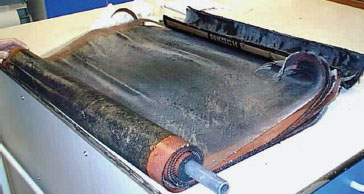
▲Figure 2. This membrane is covered with a black biofoulant.
The bane of existence for users of reverse osmosis (RO) membrane systems (Figure 1) is controlling membrane fouling from microorganisms. Autopsies of 150 membranes found that all of the membranes had some degree of membrane biofouling (Figure 2) (1). Forty-nine had microbial colony densities greater than 105 CFU/cm2, which was the direct cause of the membranes’ performance decline. Biofouling was a contributing factor to the performance decline of the other 101 systems.
Biofouling is the irreversible adhesion on a membrane of microorganisms and the extracellular polymers (ECPs, i.e., biofilm) that they produce. The process of adhesion involves three steps (2):
- bacterial adhesion, which can become irreversible in just hours, even without nutrients present
- micro-colony formation
- biofilm maturation and the formation of ECP, which protects the bacteria from biocides, flow shear, and predators.
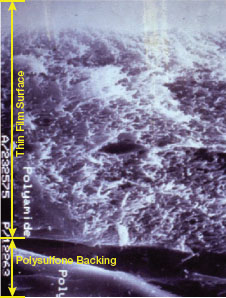
▲Figure 3. The rough surface and hydrophobic properties of this polyamide RO membrane favor bacterial adhesion.
Properties of membranes that favor adhesion and biofilm formation include:
- surface roughness (Figure 3) — the rougher the surface, the more adhesion
- surface charge — the more neutral the charge, the more adhesion of bacteria (which are negatively charged)
- hydrophobicity — the more hydrophobic the membrane, the more adhesion.
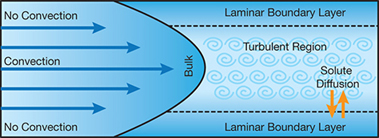
▲Figure 4. Because there is no convection in the concentration polarization layer on the surface of a membrane, dissolved nutrients and suspended solids can build up and promote bacterial growth.
Biofilm adhesion is also promoted by dissolved nutrients in the laminar boundary layer next to the membrane, which is called the concentration polarization layer (3). Because no convection occurs at the membrane surface, dissolved and suspended solids, including nutrients, build up (Figure 4).
Once a biofilm forms, it protects the underlying bacteria from disinfecting chemicals and flow shear forces that could disrupt the film.
Aerobic bacteria in seawater, brackish water, and wastewater systems, and anaerobic bacteria (e.g., iron- and sulfate-reducing bacteria) in wells, can all cause membrane biofouling. Reverse osmosis membranes used in recycle/reuse processes are exposed to an even broader range of microbes, including aerobic, anoxic, and anaerobic species.
The presence of biofouling can be indicated by (4):
- higher differential pressure, which can be evident within a few days of inoculation
- lower membrane flux, which may or may not occur prior to the change in differential pressure
- higher operating pressure required to maintain the product flowrate
- localized scaling in areas of low flow velocity caused by uneven growth of colonies, which can happen before any appreciable increase in differential pressure, particularly in spiral-wound membranes.
Techniques to address biofouling
The objectives of biofouling treatment methods are to kill the microbes; remove microbes and dead microbial bodies (which can become food for new growth); prevent adhesion, propagation, and biofilm maturation; and remove nutrients that foster microbial growth. Techniques to accomplish these objectives include:
- membrane surface modification
- modification of the bacterium and/or organic nutrient source
- disinfection, removal, or sterilization of the microbes.
Techniques to modify membrane properties, such as roughness, charge, or hydrophilicity, include coating the membrane and using a membrane made of a different polymer to minimize bacterial adhesion. Antimicrobial nanoparticles, such as silver, titanium dioxide, and carbon nanotubes, incorporated into membranes can help to limit adhesion.
Bacterium modification and disinfection reduce the concentration of viable microorganisms in the feedwater flowing to a membrane. The three basic methods of bacterium modification and disinfection are physical, thermal, and chemical.
Physical disinfection techniques include ultraviolet (UV) radiation, membrane filtration (microfiltration [MF] and ultrafiltration [UF]), and sand filtration. These techniques either modify the bacterium itself to hinder reproduction (UV) or remove bacteria according to particle size (MF, UF, and sand filtration). These methods, however, can be capital-intensive and do little to address biofilm once it has formed.
Other physical approaches are not yet commercialized, including electrochemical, ultrasonic, and thermosonication techniques.
During electrochemical treatment, a microbial suspension passes between two electrodes, which subject the bacteria to a pulsed electric field. The field disrupts the cell wall, killing the bacteria (5).
Ultrasound (sonication) is a chemical-free process that causes cell disruption by inducing cavitation in a solution. Bubbles form and break, generating turbulence and pressure differences that can rupture the microorganisms (5).
Sonication can also be combined with high temperatures (48°C) to rupture microorganisms, in a process called thermosonication. This technique is capable of damaging cells to the point that they are unable to adequately generate extracellular polymeric substance (EPS) on an RO membrane, forming fewer and less-aggregated colonies than in untreated water (5).
Thermal processes are generally not applicable to standard RO membranes, which have a stability limit of 45°C — well below the sterilization temperature required for thermal deactivation. High-temperature membranes intended for food/beverage and pharmaceutical applications typically tolerate temperatures up to 90°C for short periods of time (usually during disinfection and cleaning).
Chemical biocides are used to treat feedwater to membranes systems as well. The efficacy of a biocide depends on:
- the type of biocide and its mechanism of attack
- the concentration of the biocide; higher concentrations typically provide greater efficacy
- the occurrence of side reactions that generate inert compounds
- the presence of compounds other than microorganisms, such as organics, that compete for the biocide chemical
- the pH of the solution
- the temperature; higher temperatures improve efficacy
- the residence time of exposure; longer exposures increase efficacy
- the type of microorganisms present
- the growth state of the microorganisms
- the status and maturity of the biofilm.
Biocides are classified as oxidizing or non-oxidizing. Of the oxidizing biocides, chlorine is most commonly used for membrane applications because it is easy to use, widely available, and able to deactivate most pathogenic microorganisms quickly (3). However, because chlorine tends to form disinfection byproducts (DBPs), such as trihalomethanes (THMs) and haloacetic acids (HAAs), other biocides are increasingly being employed, including chloramine, chlorine dioxide, ozone, bromine, iodine, 1-bromo-3-chloro-5,5-dimethylhydantoin (BCDMH), peroxide/peracetic acid, potassium permanganate, copper sulfate, sodium bisulfite, dichloroisocyanurate (DCC), 2,2-di-bromo-3-nitrioproprionamide (DBNPA), and isothiazolones.
This article limits the discussion of physical and chemical RO membrane disinfection techniques to those summarized in Table 1.
Table 1. Physical and chemical techniques are used in pretreatment systems to control microorganism growth. Adapted from (6). | ||||
Method | Technique | Advantages | Limitations | Relative Cost* |
Physical | Ultraviolet(UV) |
|
| $$$$ |
Chemical | Chlorine |
Would you like to access the complete CEP Article?
No problem. You just have to complete the following steps.
You have completed 0 of 2 steps.
-
Log in
You must be logged in to view this content. Log in now.
-
AIChE Membership
You must be an AIChE member to view this article. Join now.
Copyright Permissions
Would you like to reuse content from CEP Magazine? It’s easy to request permission to reuse content. Simply click here to connect instantly to licensing services, where you can choose from a list of options regarding how you would like to reuse the desired content and complete the transaction.