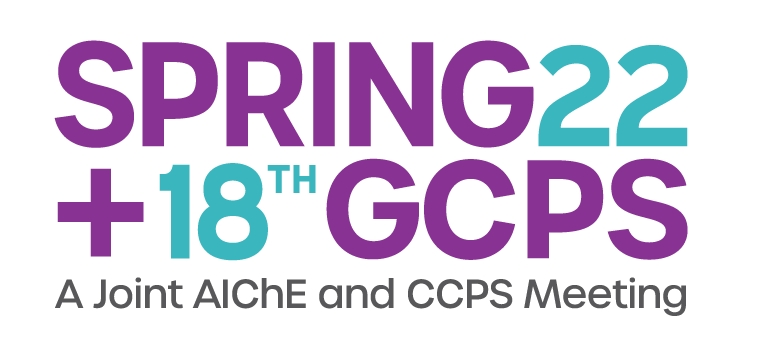
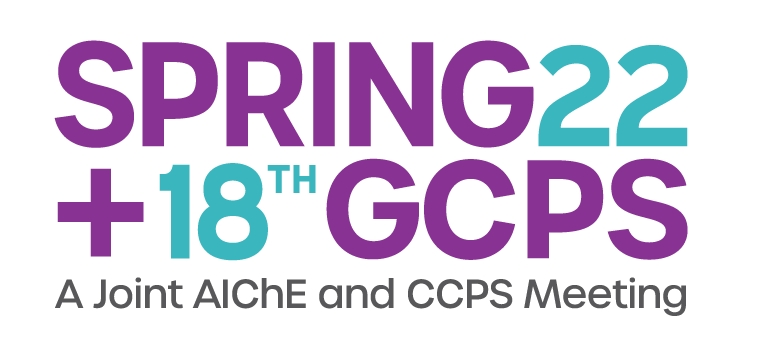
The industry is facing a serious threat about methane emissions. The topic was addressed at COP26 Summit and the methane emissions strategy should be published to finalize the EU Green Deal vision by the end of 2021.[1] Decentralized methane monetization solutions will significantly contribute to the mitigation of the issue.
The energy content per carbon number in methane is more than 20-30 % higher than in products of a Gas-to-Chemicals process. The smart utilization of that excess of energy shows a significant potential for decarbonization of an existing industrial platform.
There are three principally different ways to use the energy: autothermal oxidative conversion, electrification with either production of hydrogen or co-processing with CO2, or utilization of selective oxidation (soft oxidants or advanced reactor and catalyst concepts to limit the overoxidation).
Autothermal conversion of natural gas offers a substantial potential for decarbonization of chemical and refining industry via avoidance of GHG emissions and CO2 circularity inside assets. Co-processing of CO2 in oxidative dry/wet conversion of methane is one of the examples, which provides an efficient solution for CO2 utilization. Upgrading of the effluent will result in chemicals and hydrogen production. A right design of those processes may lead to the overall CO2-neutral/negative gas-to-chemicals technology. An example of a such of technology could be C123 process, which is under development with participation of TotalEnergies.[2] The concept of autothermal conversion shows a great number of options and would require a development of new porous materials with dual functionalities. So, the future development in the field should go beyond the improvement of the individual process performance and must be assessed according to utilization of hydrogen, heat integration, opportunistic valorization of by-products (carbon), degree of electrification, energy efficiency, and a global contribution to decrease of the overall carbon footprint of the environment.
Electrification of methane-to-chemicals provides the possibility of conditions unattainable in a conventional thermochemical reactor. The latter will allow technical feasibility of the transformation and will result in differentiation for those technologies in term of higher selectivity, and simpler production slate. One should mention that the electrification of a traditional petrochemical process (e.g., a steam cracker) will be in a competition with the proven thermochemical technology in operation with many potentially cheaper alternative decarbonization options, e.g., optimization of energy consumption, electrification of compressors, cleaner fuel (H2, NH3, hythane), and CO2 capturing technologies. In contrast, electrification of a methane conversion process is the vital condition to make technology feasible and economical. So, there is a very good chance that itâs an electrified technology of methane conversion what will be the first process brought to the market with a realistic potential to compete globally with other decarbonization solutions. The electrified processes to produce hydrogen from methane may become particularly attractive due to credits for renewable hydrogen production in the future. Currently, one of the main CO2-free alternatives for a blue hydrogen production is an electrified pyrolysis of methane.[3] The thermodynamic energy minimum to extract hydrogen from methane is about seven times lowers in comparison to the state-of-the art water electrolysis. The standard reaction enthalpy of methane pyrolysis per mole of hydrogen is also lower than the standard reaction enthalpy of the combined SMR and water-gas-shift (WGS) reaction. In theory, the SMR process also could be electrified, however the reaction produces CO2 and requires CO2 capturing. The main disadvantages of the methane pyrolysis are: (i) two times higher consumption of natural gas per ton of hydrogen in comparison to a steam reforming process, and (ii) a significant carbon coproduction (necessity to handle solids). In this context, valorization of carbon produced by the process is critical for the process viability and economics. Even if a premium quality of carbon would be produced by pyrolysis of methane, only a small part of this volume would be sufficient to saturate the market. However, it is important to mention that a substitution of the hydrogen supply at a typical refinery from SMR (about 40% of the overall demand in H2 of a refinery) by methane pyrolysis will generate several times lower carbon amount than the current volume of the petcoke production on the same site. That means that in frame of a refinery, the quantity of the solid carbon to manage is comparable to the existing operation volumes and the option to displace the H2 from SMR by methane pyrolysis is potentially feasible. One of the possible solutions to the sequestration of carbon could be its utilization as a feedstock in chemical reactions with CO2, H2O, H2S, O2 or in industrial processes. As a different look at valorization of carbon in hydrogen production from methane, one can think about leaving a part of hydrogen together with carbon in the same molecule. That could be achieved in an electrified methane non-oxidative conversion process, which results in co-production of valuable hydrocarbons together with hydrogen. Such hydrocarbons one can consider as a âcarbon-sinkâ for hydrogen production but the âcarbon-sink with a very high market size and high value at petrochemicals market, which will result in a significant additional economic value for the technology. These processes are currently under development.
One of the recent topics of a significant attention is utilization of soft oxidants. Typically, the selectivity of a typical OCM process is significantly challenged by overoxidation to CO2. Other gaseous reagents such as N2O, Halogens, and S2 have been investigated to a far lesser extent as alternative, milder oxidants to replace O2. Utilizing N2O as an oxidant could conceivably lead to enhanced C2 selectivity in OCM due to its relatively mild oxidizing tendency compared to O2. However, the challenge is in synthesis/regeneration of N2O. In this context, utilization of sulfur is more attractive because a conventional Claus unit could recover the disulfur (S2) from H2S. The oxidative coupling of methane to ethylene using gaseous disulfur as an oxidant proceeds with promising selectivity.[4] However, the process co-produces a significant amount of CS2, which needs to be valorized. Regarding halogens, Br-conversion technologies are the most advanced, e.g., a Br-mediated technology to transform methane to aromatics was developed by GTC.[5] The technology shows a promising carbon efficiency and carbon footprint.
One of the future focal points could become a fast-growing production of biomethane from biogas. The use of bio-methane allows significantly reducing the carbon footprint of the energy mix. However, the biomethane could be also one of the cost-competitive sources of bio-carbon for biofuel production, including bio-jet. In addition, the biomethane is produced mainly from non-edible and non-nutritional feedstocks and remains a concentrated source of clean energy, which shows many advantages relative to wet biomass in terms of investment, purification, and logistic costs. So, despite low production volumes, the development in the area remains relevant for the decentralization of economy and biofuel production.
The upcoming trends in electrification of downstream processes together with the emerging expansion in petrochemicals (crude-to-chemicals) lighter the product slate and result in necessity to valorize many methane-containing streams (off-gases). The ambitions to reduce the GHG emissions via electrification of the chemical industry could be only achieved if the on-purpose valorization of methane rich streams will take place and the off-gases will not be sent to flare or to fire heaters for energy production. Those emission mitigations may be achieved via utilization of the fuel gas in a different way, for instance, to produce useful products and clean energy vectors. The latest will result in a significant avoidance of CO2 emissions from flaring of those gases, as well as additional room for CO2 sink from other assets. Methane-to-chemicals may contribute in decarbonization of refinery & petrochemicals complexes via CO2 avoiding from firing of off-gases, providing solutions for CO2 utilization, and supplying low carbon H2.
There is a very good prospects to successfully address âThe Methane Challengeâ with the current progress in the development of cold-wall electrified reactor, progress in the development of bi-functional stable materials, and the economic value from decarbonization of assets, which methane conversion processes could benefit. These solutions potentially allow unlocking new opportunities in sustainable transformation of light alkanes.
[1] Global voice of gas, Eliminating of methane emissions, IGU, https://www.igu.org/news/global-voice-of-gas-4/
[2] https://www.sintef.no/projectweb/c123/
[3] J. Hydrog. Energy 45 (2020), 32, 15721â15743. https://doi.org/10.1016/j.ijhydene.2020.04.100.
[4] Arinaga, A.M.; Ziegelski, M.C.; Marks, T.J. âAlternative Oxidants for the Oxidative Coupling of Methane,â Angew. Chem. Int. Ed. 2021, 60, 2-16. DOI: 10.1002/anie.202012862.
[5] Unconventional Aromatics Processes PEP Report â 300, 2018, IHS
Presenter(s)
Language
Pricing
Individuals
AIChE Member Credits | 0.5 |
AIChE Pro Members | $19.00 |
Fuels and Petrochemicals Division Members | Free |
AIChE Graduate Student Members | Free |
AIChE Undergraduate Student Members | Free |
AIChE Explorer Members | $29.00 |
Non-Members | $29.00 |