Sections
Large investment into sustainable technologies is required to decarbonize the chemical industry. Climate-aligned finance will be a key driver for implementing decarbonization measures.
The chemical industry is as diverse as the materials and processes it involves. However, society depends heavily on a few molecules that enable most chemical value chains and provide the necessities for our daily life, from water, food, and pharmaceuticals to computers, cars, and buildings. These essential molecules are commonly referred to as basic chemicals; they include ammonia, ethylene, propylene, methanol, and aromatics — benzene, toluene, and xylene (BTX). The production of these basic chemicals dominates the chemical industry by production volume, investment, energy consumption, and carbon emissions (Figure 1) (1). The basic chemicals category accounts for around 60% of the chemical industry’s energy consumption (2) and 75% of direct and energy-related greenhouse gas (GHG) emissions (3).
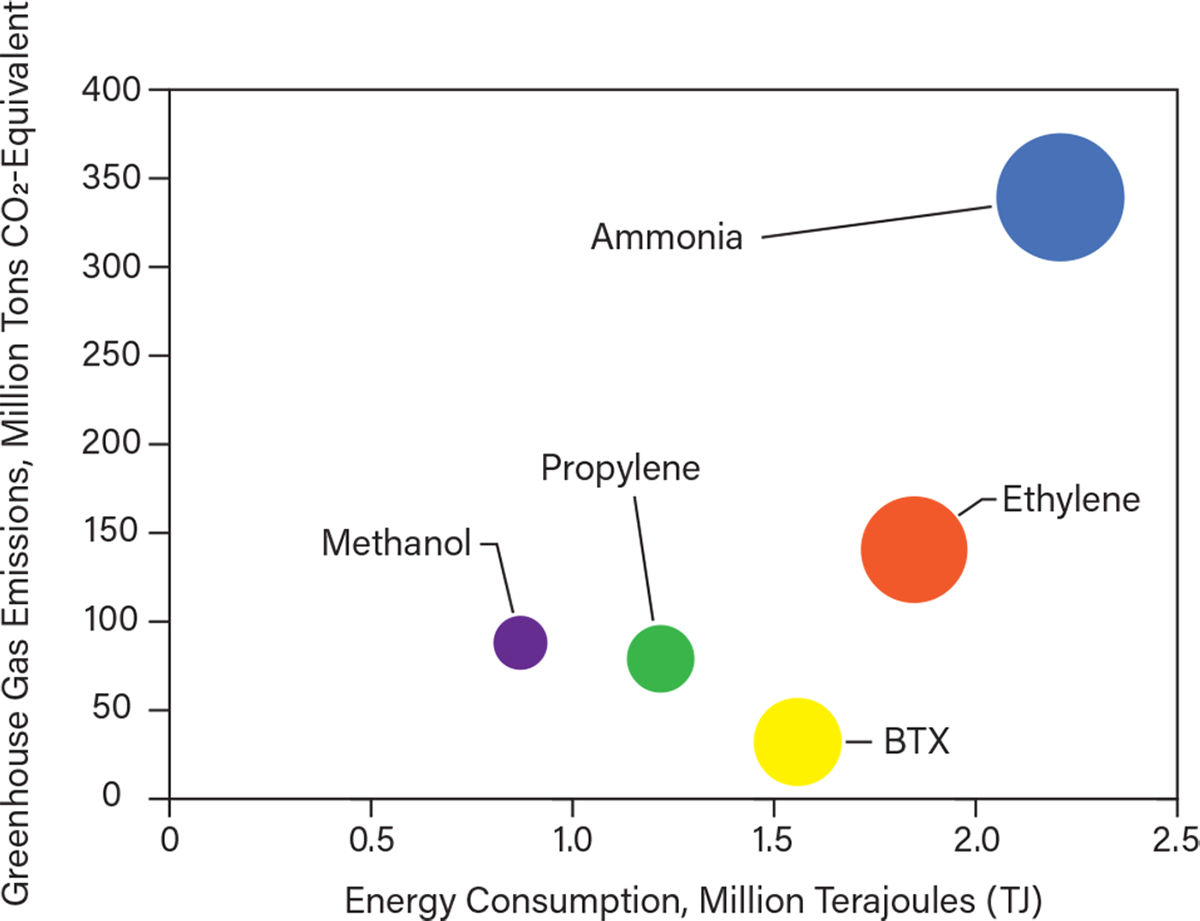
▲Figure 1. The chemical industry’s energy consumption and greenhouse gas (GHG) emissions are largely due to the production of only a few basic chemicals, which include ammonia, ethylene, propylene, methanol, and aromatics — benzene, toluene, and xylene (BTX). The sizes of the bubbles shown above are relative to production volume. Source: Adapted from Ref. 1.
The main reason for the high GHG intensity of chemical production is that it relies on the use of fossil resources such as coal, crude oil, and natural gas not only as a fuel but also as a feedstock. In addition to combustion, emissions are released from a variety of chemical reactions involved in the process technologies. Due to the large volumes of these basic chemicals produced annually, the use of fossil fuels as a raw material and energy source, and the direct process-generated emissions, the chemical industry is considered a hard-to-abate sector. However, future investment in the sector needs to be directed toward decarbonization to achieve net-zero targets and cap the global average temperature rise at 1.5°C. In response to the demands for a lower carbon future for the chemical industry, several chemical companies have announced their targets for reducing GHG emissions, whether it be maintaining certain reference emission levels or aiming for carbon neutrality (i.e., net zero) by 2050.
This article introduces the Basic Chemicals Criteria. The Climate Bonds Initiative developed this first-of-its-kind criteria to be the standard for climate-aligned bonds invested in decarbonization measures and projects in the basic chemicals industry. As part of the criteria development process, experts and professionals from academia and research organizations convened to advise on low-carbon requirements for green bonds in the basic chemicals industry. Included in this conversation was an industry working group with representatives from industry associations and investors, who offered feedback on the applicability of the criteria. After an extensive approval process, the final version of the criteria was launched in April 2022. A full digital version of the criteria is available at www.climatebonds.net/standard/basic-chemicals(4).
Green bonds for financing decarbonization
The sustainable finance market is rapidly growing and demonstrating its crucial role in the fight against climate change. Labeled bonds are fixed-income financial instruments that aim to promote some form of beneficial outcome. The three main categories are social, sustainability, and green bonds, which are used depending on the type of project and the expected outcomes. Green bonds are issued to investors by governments, multinational banks, or corporations to finance projects aimed at mitigating or adapting to the effects of climate change. The issuing entity guarantees to repay the bond over a certain period of time, in addition to either a fixed or variable rate of return (5), and repayments paid back to the investor are the proceeds earned from the project. The green bond label helps investors identify climate-aligned projects even if the investors themselves have limited resources for due diligence.
Traditional sources of capital, such as governments and commercial banks, are insufficient to fill the investment gap to decarbonize the economy, mainly due to the inherent structural characteristics of these institutions, the perceived risks of investing in green technologies, and the need for financial markets that have sufficient capacity and are dedicated to sustainability goals. Investors such as pension and sovereign wealth funds are seen as critical players in fulfilling these financing needs. Bonds are appropriate investment channels for these investors, given that bond paybacks are assured and bonds have low risk and long-term maturities.
A green bond is used to finance or re-finance projects or expenditures that address climate change. Such bonds can be given a second-party opinion or be verified or certified by entities such as the Climate Bonds Initiative, which use certification standards to give certainty and ensure that a project or expenditure meets the necessary qualifications to be labeled a green bond. Green bonds are in high demand and can help issuers attract new types of investors.
Currently, most investments in the chemical industry come from the private sector; investment portfolios are managed primarily by the International Finance Corp. (IFC) and other multilateral financing institutions (MFIs) (6). The chemical industry’s projects are part of MFI’s GHG accounting and reporting. MFIs encourage their clients to include resource and energy efficiency performance in their criteria for investments; nevertheless, there remains a lack of standard for GHG emissions for chemical plants that would be critical to determine whether a project can be financed using a green bond.
Globally, it is estimated that decarbonization of the chemical and petrochemical industry will require $75 billion for new chemical plants and energy supply (7). In Europe, the European Union (EU) allocated more than 750 billion euros in the form of a stimulus package for investments in sustainable technologies, including Li-ion batteries, green hydrogen production and storage, biorefining, and plastics recycling (8). Some of these funds will be granted to the chemicals sector. At the entity level, private companies are also allocating capital to fund climate projects, including BASF, the largest chemical company in Germany, which plans to invest up to 1 billion euros by 2025 to reach its climate target presented in 2021. BASF additionally plans to contribute another 2–3 billion euros by 2030 (9).
Recently, some chemical companies started to establish green finance frameworks to issue green finance instruments for sustainable projects that benefit the environment and society. Kaneka Corp., a Japanese chemical manufacturer, issued a green bond in 2019 to finance the construction and research and development (R&D) costs of new biodegradable polymer production facilities. In 2020, BASF issued a green bond with a volume of 1 billion euros and a term of seven years. In France, Arkema issued its first green bond to finance a new production facility to produce bio-based polyamide. Table 1 summarizes the green bonds issued in the chemical industry so far.
According to Bloomberg, the chemical industry has issued almost $14 billion in loans (2018–2020), linked primarily to carbon reduction targets. However, this constitutes less than 2% of the $555 billion of all loans issued to improve environmental sustainability. Examples of companies that have received notable loans for the purpose of climate change mitigation include Air Liquide and Solvay (10).
Which chemicals should be decarbonized first?
Because of the variety and number of chemical products, setting standards for all of them is not feasible. The chemicals sector requires a categorization that allows the highest emitters and most energy-intensive production processes to be addressed.
The EU taxonomy criteria for chemicals divide basic chemicals into organic chemicals, inorganic chemicals, plastics, and fertilizers (11). Alternatively, the International Energy Agency (IEA) focuses on two categories: basic chemicals and “the rest” (2). In 2013, a study published by the Joint Research Centre (JRC) and the European Commission assessed 26 essential chemical compounds, covering 75% of total energy use and over 90% of the GHG emitted by the chemical sector (12). The Inter-American Development Bank (IDB) includes chemicals such as ammonia, olefins, methanol, and some intermediates such as styrene and plastics (polyethylene and polypropylene) as basic chemicals. Indeed, categorization and the products included in the basic chemicals sector vary by jurisdiction, geography, organization, or entity. Despite this variation in certification and regulatory criteria, the Climate Bonds Initiative’s criteria selected basic chemicals based on a value chain approach, wherein the initial building blocks of the chemical industry are addressed first. Thus, the following list of chemical products informed the development of the Climate Bond Initiative’s criteria:
Inorganic basic chemicals:
- ammonia
- chlorine
- disodium carbonate/soda ash
- nitric acid
- carbon black.
Organic basic chemicals:
- high-value chemicals: acetylene, ethylene, propylene, butadiene
- aromatics (BTX)
- methanol.
Since ammonia and the basic organic chemicals shown in Figure 1 represent the largest production by volume and the largest contribution to GHG emissions from the chemical industry, the chemical industry’s decarbonization pathways and policies have focused largely on these chemicals. Reducing emissions from the production of these chemicals has the highest potential for climate change mitigation, both within their own production processes and their downstream value chains. One example of this approach in practice is the EU’s taxonomy, which already requires emissions benchmarks or qualitative criteria in order for the production of these chemicals (with the exception of methanol) to be considered environmentally sustainable.
Decarbonization of basic chemicals is difficult mainly due to the high process temperatures or direct process emissions produced by the chemical reactions. Table 2 presents the main technical challenges for the decarbonization of basic chemical production processes and examples of decarbonization options.
Decarbonization options aim to replace fossil fuels such as coal and natural gas with renewable sources or to use waste heat to produce energy. One of the most important technologies is electrically heated processes, such as steam crackers, that produce basic chemicals such as ethylene, propylene, and butadiene. Other examples include water electrolysis and methane pyrolysis, which are also being investigated for their use in producing low-carbon hydrogen, a required chemical for producing ammonia and methanol. Carbon capture and CO2 reuse are also common decarbonization technologies considered in corporate chemical industry decarbonization plans.
Large-scale decarbonization projects will require investment, which will likely come from the financial sector. The financial sector requires certainty that funds are applied to impactful and legitimate mitigation measures based on scientifically grounded approaches and effective strategies. Analytical frameworks and certification tools are necessary to identify viable decarbonization pathways. This standard framework enables the financial sector to prioritize projects according to their alignment with the goals of the Paris Agreement and to minimize the risk of assets being stranded. The Climate Bond Initiative’s Basic Chemicals Criteria provide this framework.
Emission classifications
There are three different scopes of emissions, as defined by various protocols and standards used by different companies and institutions, that describe the source of the emission and how directly those emissions relate to the initial chemical process (Figure 2). These scopes include:
- Scope 1 emissions. Scope 1 emissions are all direct emissions from the production processes, including emissions generated during the reactions and emissions from fuel combusted for on-site energy generation.
- Scope 2 emissions. Scope 2 emissions are indirect emissions from the energy sources, i.e., emissions deriving from the electricity, heat, or other energy forms imported from off-site. Note that not all standards include Scope 2, and thus, a requirement for renewable electricity is included in the Basic Chemicals Criteria as an additional criterion.
- Scope 3 emissions. Scope 3 emissions include all other emissions from the supply chain, divided into upstream and downstream emissions. Upstream refers to the activities for supplying the raw material and energy inputs, and downstream refers to the subsequent use of a product, the product’s end use, and the product’s disposal.
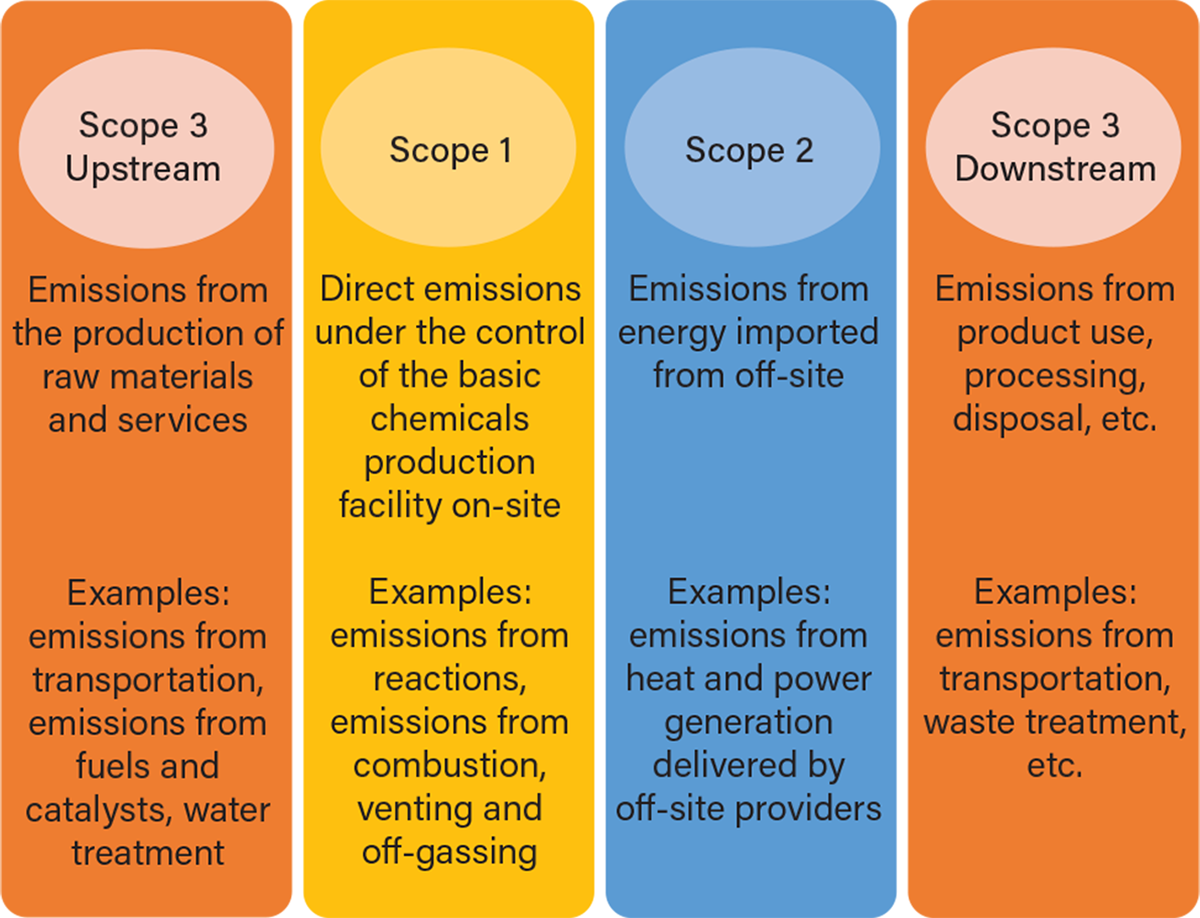
▲Figure 2. The scopes of emissions describe the emission’s source and how directly those emissions relate to the initial chemical process. The order of the scopes shown above represents the order in which emissions are generated before, during, and after the chemical’s production, with Scope 3 upstream emissions occurring before the chemical is manufactured and Scope 3 downstream emissions deriving from the product’s use, processing, or disposal.
Note that Scope 1 emissions are produced on-site and under the facility’s control. Scope 2 emissions are from imported energy and are considered indirect as they are released off-site. For example, in some processes, hydrogen is produced inside the basic chemical facility; therefore, the associated emissions are under its control, and the emissions from hydrogen production should be accounted for. If the hydrogen is purchased from off-site, then the associated emissions are under Scope 2, as in the case of purchased electricity. Also, note that in the case of carbon capture and sequestration (CCS), the emissions from the capture, transportation, and storage activities should be accounted for, while the amount of CO2 captured is subtracted to calculate the final carbon intensity of a product. Similarly, if the venting and off-gases released into the atmosphere contain any GHG, they should be accounted for in the GHG accounting.
Scope 3 is more complex because the emissions are released upstream and downstream from the value chain. Chemical producers may not even know where and how their product is used. Similarly, the supply of raw materials and utilities may come from different sources and suppliers, which might produce varying levels of emissions. Thus, setting criteria for addressing Scope 3 is challenging, and only certain qualitative guidance and disclosure of measures are usually considered when evaluating a process’s eligibility for sustainable financing.
Setting criteria for certification
The Basic Chemicals Criteria can be used to certify and verify the positive environmental impact of assets, such as basic chemicals production plants, as well as the following financial instruments:
- use of proceeds (UoP) bonds, or debt instruments allocated to specific projects
- sustainability-linked bonds (SLBs), or debt instruments for general corporate purposes, not specific projects. For SLBs, the objectives of the bond are measured through predefined key performance indicators (KPIs) and assessed against predefined performance targets.
The Basic Chemicals Criteria are divided into three categories, which are designed to ensure different aspects of an asset or project’s efficacy and resilience. Each component needs to be satisfied for assets to be eligible for certification by the Climate Bonds Initiative:
- Mitigation criteria. Criteria describing the necessary decarbonization and energy-intensity reduction benchmarks that facilities or processes must meet in order to be considered sustainable and, thus, eligible for capital investment financial instruments.
- Adaptation and resilience cross-cutting criteria. Criteria requiring projects or facilities to plan adaptation and resilience measures for events or scenarios produced by climate change (e.g., extended drought seasons, heavier storms, stronger winds, etc.); provisions for addressing other environmental impacts and disclosure of information related to environmental impacts are also incorporated and can be found in the Basic Chemicals Criteria.
In setting the criteria, it was important to differentiate between existing operating assets transitioning toward low-carbon production processes and those financed as brand-new assets. Although criteria apply to both types of facilities, additional requirements are set depending on which category an asset falls under (4). There are three main reasons for this: to ensure emissions reduction over time, to prevent stranded assets, and to prevent carbon lock-in, which is the tendency for certain carbon-intensive technologies to persist over time because they are known and trusted.
Preventing carbon lock-in. Carbon lock-in perpetuates high-carbon emitting processes for many decades and creates the inertia that prevents the adoption of lower-carbon alternatives. With chemical plants typically having an average operating life of 30 years, new plants built with conventional technologies will likely not contribute to carbon-emission-reduction goals. Therefore, the Basic Chemicals Criteria require new facilities to use low-carbon approaches to prevent potential carbon lock-in. A study by the Stockholm Institute shows that the potential carbon lock-in of chemical plants could be up to 5 Gt CO2 if new plants are designed using current commercial technologies and configurations (13). For examples of high-carbon traditional technologies, Table 1 includes the conventional, or business-as-usual, technologies for the basic chemicals.
Preventing stranded assets. As efforts to address climate change develop and fossil assets are kept in the ground, any chemical asset reliant on fossil energy and feedstock (e.g., pipeline, chemical refinery) may find it increasingly hard to access reliable source material, ultimately becoming a stranded asset. To prevent stranded assets and better align new facilities with a low-carbon future, constraints for technology and feedstocks are included in the mitigation requirements.
Mitigation criteria
Criteria for production facilities. The overarching goal behind all mitigation criteria is that projects and processes meet emissions or energy-intensity-reduction benchmarks set for each product, as shown in Table 3 (4). Some of the 2022 benchmarks were adopted from the goals of the EU’s Emissions Trading System, which were informed by best-in-class production facilities. However, for specific products, such as olefins, more ambitious benchmarks were proposed, considering the high share of emissions from these products and the large volume of downstream products these chemicals are used to produce. Additionally, facilities that produce these high carbon-emitting chemicals must reduce emissions over time to be close to net zero by 2050. The trajectory laid out by the Climate Bond Initiative’s criteria is aligned with a decarbonization pathway for the chemical industry proposed in 2021 by Teske et al. at the Univ. of Technology Sydney (14). This pathway is clearly aligned with the cross-sectoral pathway from the Science Based Targets initiative (SBTi) (15), an initiative that provides guidance for companies and sectors looking to set science-based climate change mitigation targets, as well as the pathways for direct CO2 emissions estimated by IEA in a Sustainable Development Scenario (SDS) (Figure 3). Notice that the pathway shown also converges to the direct CO2-only emissions of the IEA scenario. Further, depending on the production technology, feedstock, or fuel, specific requirements were defined for fossil-fuel-based production, including methane-leak monitoring, reporting, and verification mechanisms.
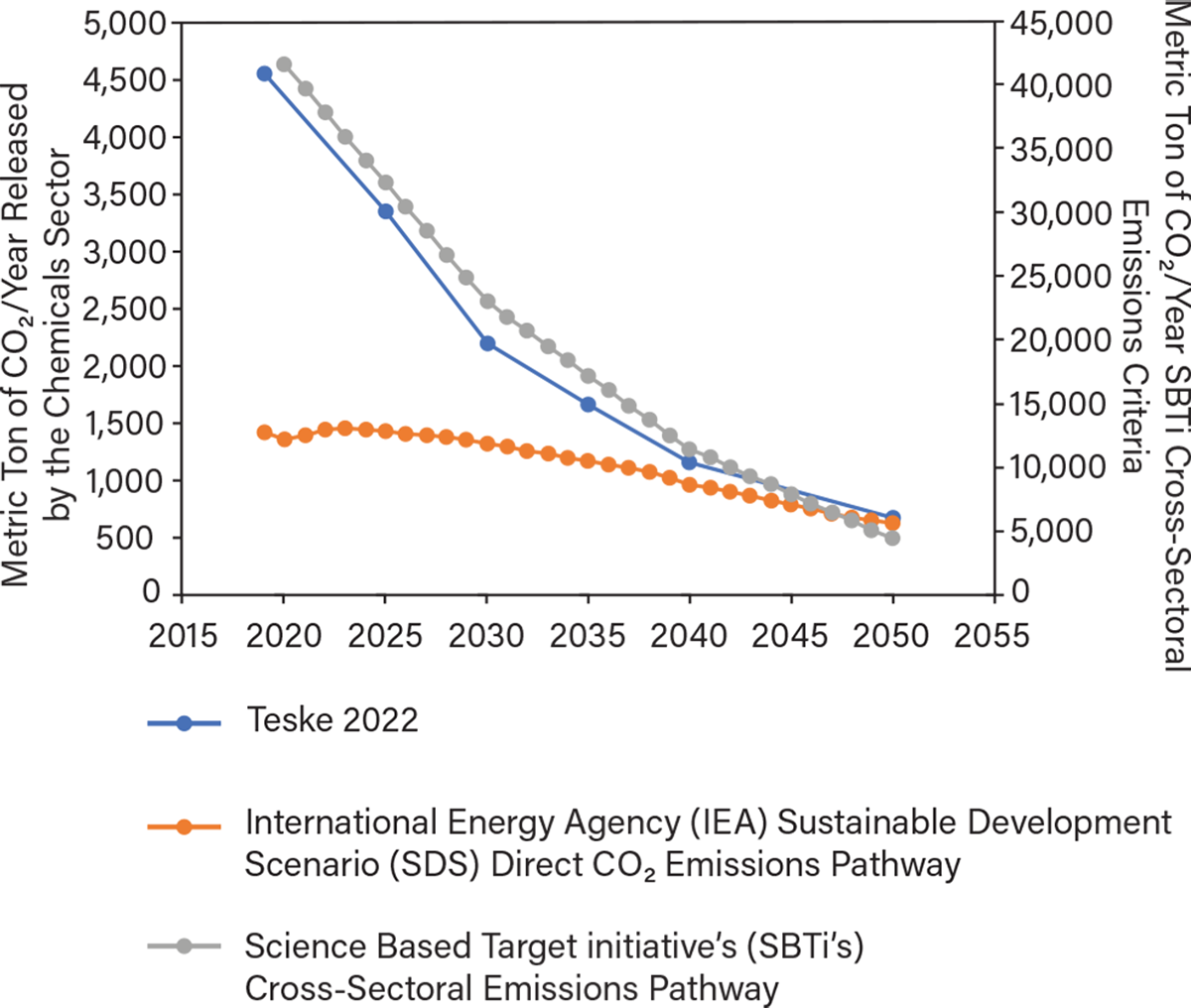
▲Figure 3. Many companies and institutions have plans and pathways to significantly reduce carbon emissions by 2050. These plans include the International Energy Agency’s (IEA’s) Sustainable Development Scenario (2), the Science Based Target initiative’s (SBTi’s) cross-sectional pathway (15), and the pathway laid out by Teske et al. at the Univ. of Technology Sydney (14). The carbon emissions projected to be released by the chemical industry according to each pathway are measured along the left axis, while the SBTi’s analysis of cross-sectoral carbon emissions is comparatively shown on the right.
Criteria for decarbonization measures. During the initial drafting of the Basic Chemicals Criteria, the Technical Working Group discussed various decarbonization strategies. They considered it pertinent to set specific criteria for the decarbonization measures that showed the most promise for decarbonization. The guiding principles in setting specific criteria for decarbonization measures were:
- consistency with the level of ambitions required by the Paris Agreement for a low-carbon future, including the need to transition from fossil fuels to alternative sources of energy and feedstock
- ability to identify additional measures that need to be used in combination to become eligible. For example, if a company wants to use natural gas, it may need to use CCS, employ leakage detection technology, and monitor carbon emissions
- consistency with existing climate bond criteria for directly related sectors. This means that when implementing any of these measures, care should be taken to not jeopardize the primary goal of reducing emissions and the mitigation objectives of other sectors. For example, the generation of low-carbon hydrogen feedstock cannot be at the expense of using high-carbon electricity for its production via an electrolytic process. Similarly, the CO2 captured to mitigate the impact of burning fossil fuels cannot then be used to excuse further extraction of more fossil fuels through enhanced oil recovery.
Decarbonization measures include energy efficiency projects, electrification of processes, fuel and feedstock substitution, and carbon capture and storage. The requirements aim to address potential negative environmental impacts and avoid greenwashing, which is the practice of using publicity and marketing to create the false impression that a product or company is producing an environmental benefit.
Criteria for entities and SLBs. Two levels of entity and SLB certification are available: aligned certification for entities already on the pathway to being close to net zero by 2050 and transitioning certification for entities not aligned at the time of certification but with a transition plan to be close to net zero by 2050.
Adaptation and resilience criteria
The Basic Chemicals Criteria also promote project resilience and adaptability when faced with potential climate change risks. Projects must demonstrate that they are prepared for and able to recover from the impact of climate change.
Potential risks include negative impacts to capital assets, transport and raw materials availability difficulties, and productivity and safety problems. Because of the type of hazardous substances manufactured, managed, and stored in chemical facilities, climate change risks could lead to severe consequences.
At the root of the Climate Bonds Initiative is the principle that processes should be adaptable and resilient, and the adaptation and resilience (A&R) component of the criteria adapts this principle to meet the needs of the chemical industry (16). These criteria require that facilities conduct a risk management plan (RMP) for the chemicals in the U.S. Environmental Protection Agency’s (EPA’s) list of regulated substances (17). This checklist of requirements ensures that certifiable projects:
- understand the context and clearly define boundaries that may be directly affected by the operation of the facility and interdependencies with other value chains
- address climate risks by completing climate risk assessments and planning ways to reduce risk
- address resilience benefits by performing benefits assessments and assessing climate mitigation trade-offs. Benefits demonstrate that the system or measure significantly contributes to enhancing climate resilience. Potential trade-offs are positive resilience measures that may have a negative climate mitigation impact. For example, a desalination plant will help address water scarcity, but it is an energy-intensive activity.
- monitor and evaluate risks to processes
- address possible environmental impacts by implementing pollution prevention strategies.
Closing thoughts
The chemical industry will need a diverse portfolio of measures, tools, and strategies to decarbonize and bring about a low-carbon future. To help mitigate climate change, finance through green bonds and SLBs requires standards to remove risk for investors and ensure the capital that will allow the development and operation of new, low-carbon technologies. The Climate Bonds Initiative’s criteria were the first of their kind, establishing clear requirements for mitigation targets and outlining direct pathways for the industry to approach net-zero GHG emissions and align with the goal of reducing global average temperature increase to 1.5°C. These criteria require future processes to be able to adapt and be resilient to threats presented by climate change. Although accurately predicting the effects of evolving technologies and a changing climate is difficult, any current or future criteria for sustainable investment must account for these challenges. However, chemical engineers applying their skills to design new low-carbon technologies and ensure compliance with emissions-reduction guidelines will be key to achieving the chemical industry’s ambitious targets in the years to come.
Acknowledgments
Climate Bonds gratefully acknowledges the Technical and Industry Working Group members who supported the development of these Criteria.
Mitigation Technical Working Group (TWG): Camilla Oliveira, Agora-Energiewende; Carina Oliveira, The Netherlands Organization of Applied Scientific Research (TNO) Energy Transition Studies; Jonathan Moncada, TNO Energy Transition Studies; Kok Siew Ng, Univ. of Oxford; Denny K. S. Ng, Heriot-Watt Univ., Malaysia Campus; Peter Levi, International Energy Agency (IEA) — Energy Technology Policy Division; Fredric Bauer, Lund Univ.; Robert Adamczyk, European Bank of Research and Development (EBRD); Gbemi Oluleye, Imperial College London — Centre for Environmental Policy in the Faculty of Natural Sciences; Thomas Rehm, AIChE’s Institute for Sustainability; and Heriberto Cabezas, Univ. of Miskolc.
Adaptation and Resilience TWG: Dale Sands, Sands Consulting Solutions; Kristin Marshall, Lux Research; Fahim Tonmoy, Griffith Univ.; and Rita Ferreira, S&P Global Research.
The Industry Working Group provided critical and useable focused consultation and feedback on the Basic Chemicals Criteria, but this does not automatically reflect the endorsement of the criteria by all members.
Literature Cited
- Schiffer, Z. J., and K. Manthiram, “Electrification and Decarbonization of the Chemical Industry,” Joule, 1 (1), pp. 10–14 (Sept. 6, 2017).
- International Energy Agency, “Energy Technology Perspectives 2020,” www.iea.org/reports/energy-technology-perspectives-2020, IEA, Paris, France (2020).
- Science Based Targets Initiative, “Barriers, Challenges, and Opportunities for Chemical Companies to Set Science-Based Targets,” www.sciencebasedtargets.org/resources/files/SBTi-Chemicals-Scoping-Document-12.2020.pdf, SBTi, London, U.K. (Dec. 2020).
- Climate Bond Initiative, “Basic Chemicals Criteria,” https://www.climatebonds.net/standard/basic-chemicals, London, U.K. (accessed Jan. 26, 2024).
- Climate Bonds Initiative, “Understanding Climate Bonds,” www.climatebonds.net/resources/understanding, London, U.K. (accessed Jan. 26, 2024).
- Suding, P. H., “Chemical Plant GHG Emissions: Reconciling the Financing of Chemical Plants with Climate Change Objectives,” No. IDB-TN-618, Inter-American Development Bank, Washington, DC (Dec. 2013).
- Saygin, D., and D. Gielen, “Zero-Emission Pathway for the Global Chemical and Petrochemical Sector,” Energies, 14 (3), pp. 1–28 (June 23, 2021).
- Bettenhausen, C., “C&EN’s World Chemical Outlook 2021,” C&EN, www.cen.acs.org/business/CENs-World-Chemical-Outlook-2021/99/i2, Washington, DC (Jan. 10, 2021).
- Nonnast, T., “BASF Presents Roadmap to Climate Neutrality,” BASF Business & Financial News, www.basf.com/global/en/media/news-releases/2021/03/p-21-166.html, Ludwigshafen, Germany (Mar. 26, 2021).
- Kane, E., “Many Global Chemicals Companies Trail on Carbon-Transition Goals,” Bloomberg Professional Services, www.bloomberg.com/professional/blog/many-global-chemicals-companies-trail-on-carbon-transition-goals (Jan. 27, 2021).
- European Commission, “EU Taxonomy for Sustainable Activities,” EC, www.ec.europa.eu/info/business-economy-euro/banking-and-finance/sustainable-finance/eu-taxonomy-sustainable-activities_en, Brussels, Belgium (accessed Jan. 26, 2024).
- Boulamanti, A., and J. A. Moya, “Energy Efficiency and GHG emissions: Prospective Scenarios for the Chemical and Petrochemical Industry,” Publications Office of the European Union, Luxembourg, Luxembourg (May 5, 2017).
- Erickson, P., et al., “Assessing Carbon Lock-In,” Environmental Research Letters, 10 (8), pp. 1–7 (Aug. 25, 2015).
- Teske, S., et al., “1.5°C Pathways for the Global Industry Classification (GICS) Sectors Chemicals, Aluminium, and Steel,” SN Applied Sciences, 4 #125 (Apr. 1, 2022).
- Science Based Targets Initiative, “Pathways to Net-Zero,” https://sciencebasedtargets.org/resources/files/Pathway-to-Net-Zero.pdf, SBTi, London, U.K. (Oct. 2021).
- Climate Bonds Initiative, “Climate Resilience Principles,” Climate Bonds, London, U.K. (2019).
- U.S. Environmental Protection Agency, “Risk Management Program (RMP) Rule Overview,” EPA, Washington, DC (accessed Jan. 26, 2024).
Copyright Permissions
Would you like to reuse content from CEP Magazine? It’s easy to request permission to reuse content. Simply click here to connect instantly to licensing services, where you can choose from a list of options regarding how you would like to reuse the desired content and complete the transaction.