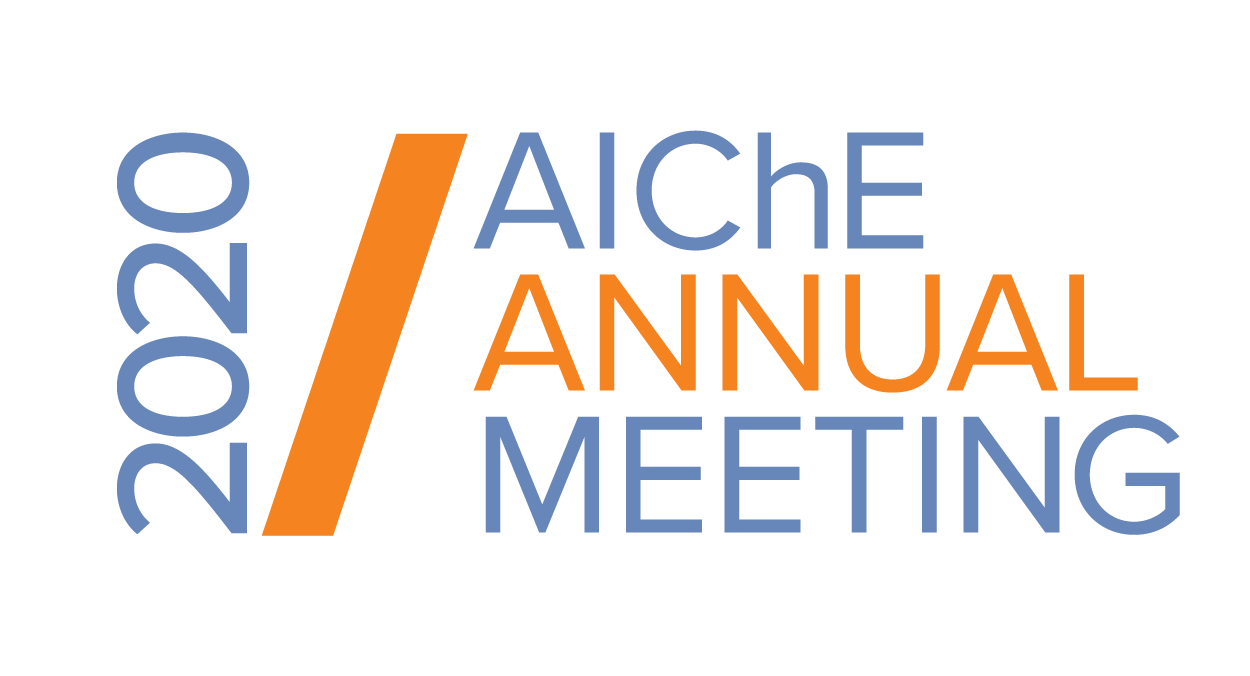
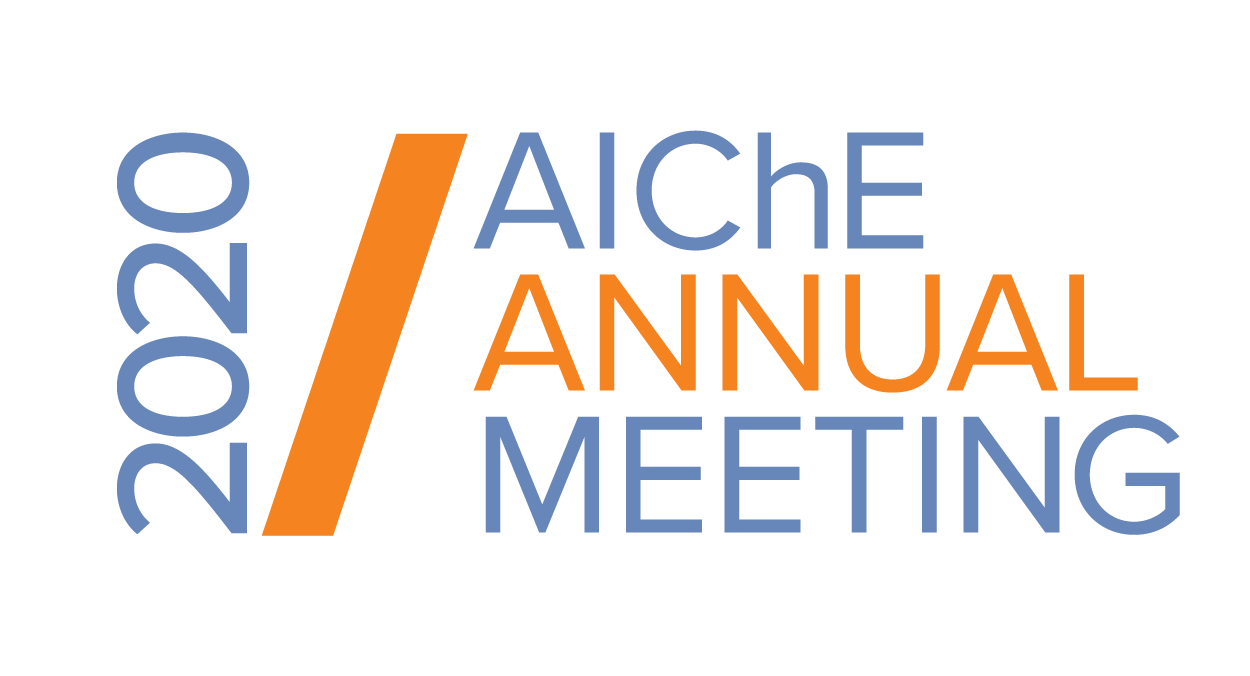
Today, TCES is at an early stage of development. Previously, several fluid phase and solid-gas reactions have been studied. Recent analyses of fluid-phase reactions show that they suffer from low energy efficiency and high cost due to the need to store reacting gases.2 However, solid-gas reactions are more promising because they require less gas storage and the products can be easily separated. Some work has been done to identify promising reaction candidates3,4 based on equilibrium temperature and energy density. However, it still remains unclear which reaction chemistry leads to the lowest storage and electricity production costs. Accordingly, the goal of this work is to (i) evaluate solid-gas reactions belonging to the oxidation, hydration, and carbonation family via optimization models and (ii) rank material properties based on their effect on energetic and economic performance of CSP-TCES plants.
To this end, we developed a general process synthesis model for CSP with solid-phase TCES systems. The model is formulated as a two-stage stochastic programming model to account for variability in daily and seasonal solar irradiance, which are represented as modes and scenarios, respectively. The model requires plant characteristics (e.g., weather, operation time, and capacity) and reaction properties (e.g., reaction enthalpy and entropy, and specific heat capacity of each component) as inputs. Daggett in southern California is selected as the plant location with 100 MW capacity and its weather data is obtained from National Solar Radiation Data Base.5 Component heat capacity, reaction enthalpy and entropy are estimated using Barin database.6 The solution of the optimization model yields design (e.g., solar field area, receiver size, reactor and storage tank sizes) and operating conditions (e.g., stream flow rates, charging and discharging duration) that leads to the minimum levelized cost of electricity production. Other performance metrics such as energy efficiency and storage costs are also computed.
We take three major steps to create a ranked list of promising reaction candidates. First, we create a comprehensive list of reaction candidates belonging to oxidation, hydration, and carbonation family based on stoichiometry. Second, to maintain computational tractability, we perform a preliminary screening, and choose the reactions with positive enthalpy and equilibrium temperatures between 400 oC and 1500 oC. Third, the model is solved for the reaction candidates obtained after preliminary screening and they are ranked based on the cost of electricity production. Furthermore, we also formulate a material optimization problem by including reacting material properties as the decision variables in the process synthesis model. This analysis provides insights in the material and reaction properties that are most suitable for CSP-TCES plants and serve as guidelines for the development of novel TCES systems.
References
1 IEA publications, Energy Technology Perspectives 2017 - Catalysing Energy Technology Transformations, 2017.
2 X. Peng, T. W. Root and C. T. Maravelias, Green Chem., 2017, 2427â2438.
3 P. Pardo, A. Deydier, Z. Anxionnaz-Minvielle, S. Rougé, M. Cabassud and P. Cognet, Renew. Sustain. Energy Rev., 2014, 32, 591â610.
4 L. André, S. Abanades and G. Flamant, Renew. Sustain. Energy Rev., 2016, 64, 703â715.
5 NREL, National Solar Radiation Data Base, https://rredc.nrel.gov/solar/old_data/nsrdb/1961-1990/, (accessed 8 April 2020).
6 I. Barin, Thermochemical Data of Pure Substances, VCH Verlagsgesellschaft, FRG., 1989.