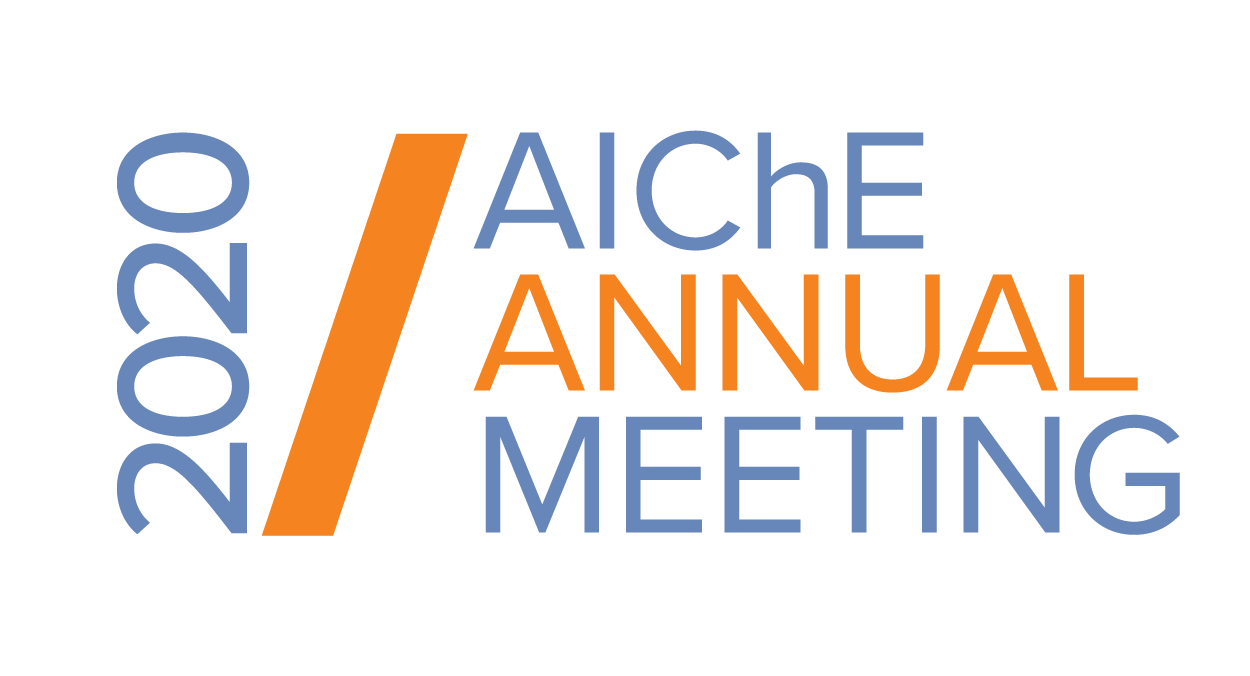
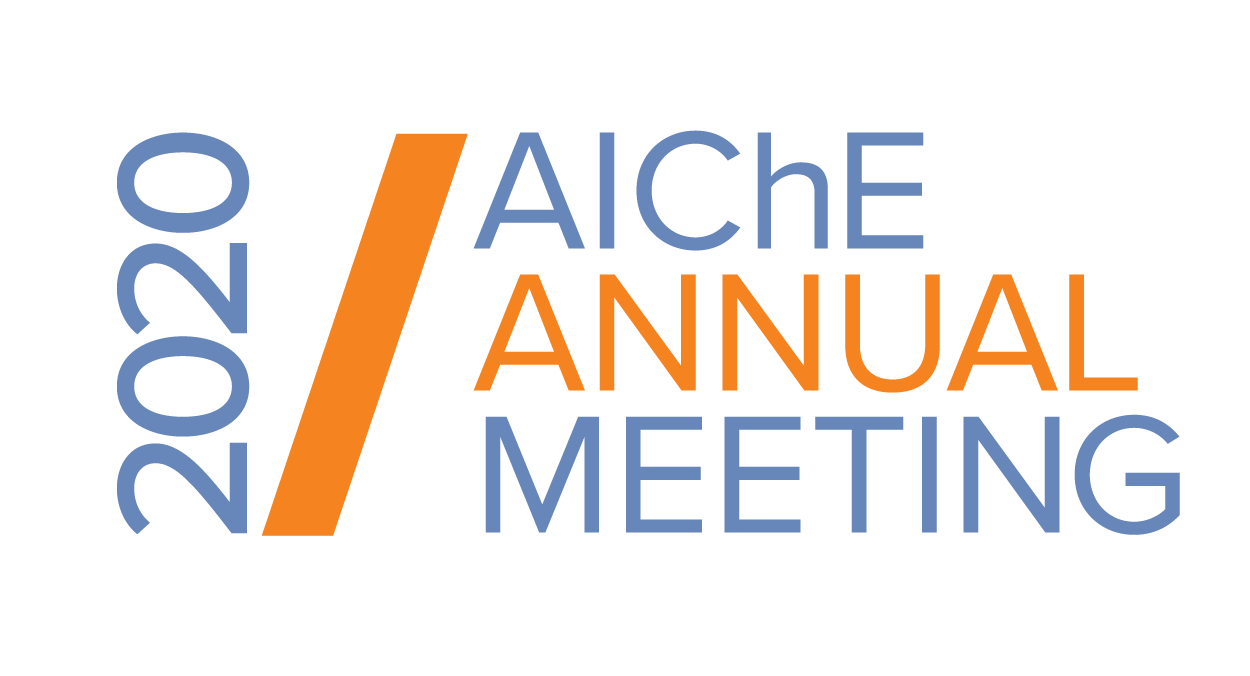
Solar electricity generation inherently suffers from intermittency and unequally distributed irradiation conditions. The conversion of solar energy into chemical energy carriers, i.e. solar fuels - which can be long term stored and transported - can tackle some of the drawbacks linked to the utilization of this renewable resource [1]. Water and/or CO2 splitting, either using concentrated solar power or though photo-chemical and photo-catalytic processes, have been intensively studied in the last decades. New materials and innovative solar reactors have been proposed, highly contributing to the development of solar fuels technologies [2,3].
Carbon dioxide is indeed a very stable molecule. Any process envisaging its transformation into fuels or chemicals requires important amounts of energy and generally implies stringent conditions in terms of temperature and/or pressure. Methane production from CO2 is not an exception. Carbon dioxide methanation, through the well-known Sabatierâs reaction (Eq. 1), implies its hydrogenation/reduction, yielding methane and water. When coupled to water electrolysis, the production of synthetic methane from CO2 is seen as a promising tool for storing off-peak renewable electricity production (power-to-gas concept).
CO2(g)+ 4H2(g) = CH4(g) + 2H2O(g) âH°25°C= -165.3 kJâmol (Eq. 1)
The Sabatierâs reaction is exothermic and thermodynamically favorable at ambient temperature and pressure. However, it is strongly hindered by its sluggish reaction kinetics. Even in the presence of a catalyst, moderate temperatures - normally over 350°C - are needed to overcome this kinetic barrier. Increasing the operating pressure up to 20-30 MPa helps controlling the formation of by products, such as CO, and catalyst deactivation.
(Fig. 1. Plasma-catalytic redox cycle in the presence of a Ni/CeZrOxcatalyst.)
Lately, we have demonstrated that the plasma-intensification of CO2methanation can lead to high methane yield under pseudo-adiabatic conditions (no external heating source) and atmospheric pressure [4-6]. A Dielectric Barrier Discharge (DBD) cold plasma was generated within two electrodes, leading to the ionization of the reactant gas mixture. In the presence of a Ni-containing CeZrOx-supported catalyst, the reaction was 100% selective to methane and no sign of deactivation was observed over 100 h operating time. The information gathered through a detailed physicochemical characterization of the spent catalyst led us to conclude that the use of a CeZrOxsupport, a non-stoichiometric oxide played a very important role in a redox cycle that is furthermore sustained by the transfer of electrons in the gas plasma [7]. In the presence of a DBD plasma, our catalyst works as a PN junction: electrons are transferred from the Ni-active sites to the Ce-cations in the support. Oxygen transfer follows, leading to continuous oxidation and reduction of both the active phase and the non-stoichiometric oxide, as depicted in Fig. 1. In the absence of the DBD plasma, the random-Brownian movement of gas molecules impedes an efficient electron transfer, the redox cycle is not completed, oxygen accumulates on the surface and the catalyst deactivates with time-on-stream.
Electron transfer is therefore a key issue in CO2 reduction or splitting reactions. Both the dielectric properties of the catalyst and its physicochemical features can therefore be tailored in order to increase the yield in the targeted products under plasma-catalytic operation. Our latest results open up the door for further research on plasma catalytic processes for CO2 recycling, offering new solutions for renewable energy storage and the production of synthetic fuels from CO2.
[1] A. Steinfeld, A. Meier, Encyclopedia of Energy, Ed. Elsevier, 5 (2004) 623-637.
[2] W.C. Chueh, C. Falter, M. Abbott, D. Scipio, P. Furler. S. M. Haile, A. Steinfeld, Science 330 (2010) 1797-1801.
[3] A. Corma, H. Garcia. J. Catal. 308 (2013) 168-175.
[4] M. Nizio, A. Albarazi, S. Cavadias, J. Amouroux, M. E. Galvez and P. Da Costa, Int. J. Hydrog. Energy 41 (2016) 11584â11592.
[5] M. Nizio, R. Benrabbah, M. Krzak, R. Debek, M. Motak, S. Cavadias, M. E. Gálvez and P. Da Costa, Catal. Commun. 83 (2016) 14â17.
[6] M. Mikhail, B. Wang, R. Jalain, S. Cavadias, M. Tatoulian, S. Ognier, M. E. Gálvez and P. Da Costa, React. Kinet. Mech. Catal. 126 (2019) 629â643.
[7] M. Mikhail, P. Da Costa, J. Amouroux, S. Cavadias, M. Tatoulian, S. Ognier, M.E. Gálvez. Cat. Sci. Technol. In press(2020). https://doi.org/10.1039/D0CY00312C.