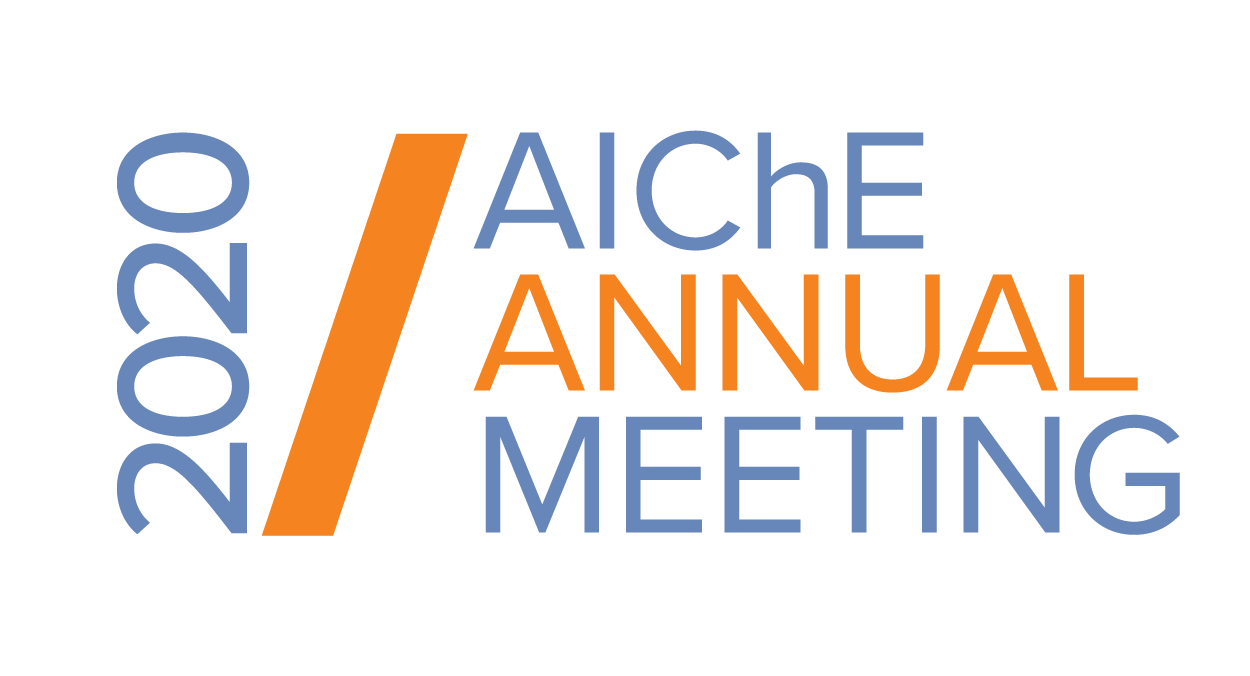
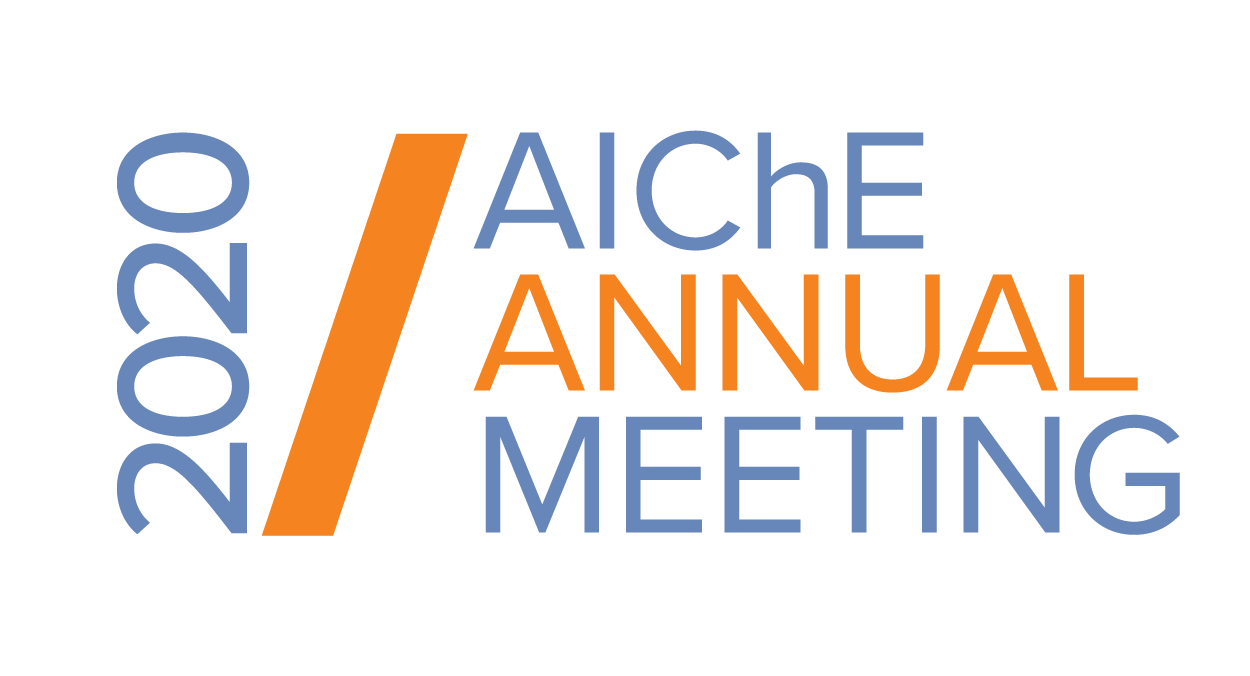
- Introduction
In the field of renewable energies, solar is the only energy source that can be exploited, with proper concentration techniques, to fulfill the needs of applications requiring thermal energy at very high temperature levels, e.g. higher than 1â000°C, such as thermochemical syngas production or industrial process heat (i.e., cement pyroprocessing). However, the maximum operating temperature of commercial solar receivers is currently lower than 873 K due to major limiting factors such as: heat transfer fluids (HTF), materials, and heat losses [1]. The Swiss company Synhelion SA is developing a new generation of solar receivers, for large-scale installations, suitable to operate at temperature levels higher than 1â500 °C [2]. The experimental demonstration will be detailed in [3]. As opposed to conventional commercial receivers, which mainly rely on indirect heating of the HTF, the proposed solution is based on the direct absorption of thermal radiation by a gaseous HTF. The latter must have specific spectral properties: it has to be almost transparent to the high-radiation intensity wavelengths of the solar spectrum and, at the same time, mostly opaque (i.e. strong absorption bands) in the wavelength range of thermal radiation. Water vapor or carbon dioxide are among the suitable HTF candidates. In the present paper, the absorbing gas receiver concept will be introduced along with a detailed description of the numerical model developed to evaluate its performance. Various simulations campaigns were carried out with the aim of evaluating the effect of important parameters, such as: (i) HTF mass flow rate, (ii) HTF entrance angle and (iii) gravity, on the receiver thermo-fluid dynamics behavior. The major findings obtained will be presented and discussed.
- The Synhelion absorbing gas solar receiver
The Synhelion absorbing gas receiver, schematically depicted in figure 1a, is a cavity-type receiver. Concentrated solar radiation enters the receiver from the windowed aperture, travels along the whole cavity length to be then absorbed by highly-absorptive surfaces located at the back of the cavity. This, in turn, causes the temperatures of these surfaces to sensibly increase thus re-emitting longer wavelength thermal radiation into the cavity. At the same time, the HTF flows also from the aperture towards the back of the cavity absorbing the thermal radiation emitted by the absorptive surfaces. As a result, the temperature of the HTF increases, as it flows through the cavity, reaching the maximum value as it approaches the innermost section in the vicinity of the receiver outlet section. The fact of being mostly opaque in the wavelength range of thermal radiation, also enables the HTF to effectively shield the absorptive surfaces from aperture minimizing hence the radiative heat losses towards the external environment.
- Numerical model development and validation
A computational fluid dynamics (CFD) model was developed, exploiting Fluent code from ANSYS, with the twofold aim of clearly understanding the physical phenomena, as well as their interactions, occurring into the system and creating a useful tool for assisting the design phase of the receiver. From the numerical modeling standpoint, the major challenge is that radiative heat transfer in participating media has to be accounted for. Based upon a tradeoff between results accuracy and computational efforts, this heat transfer mechanism was described through the discrete ordinates (DO) method coupled with the weighted-sum-of-gray-gases (WSGG) model accounting for the variations of the participating media radiation properties.
At the time this study was conducted, no experimental data were available; the CFD model was hence validated against benchmark results of a numerical model, developed by Synhelion, based on Monte Carlo line-by-line that represents the most accurate methods to model radiative heat transfer in participating ia [4]. The validation process allowed to observe that, despite a slight overestimation of the receiver performance (of about 5%), the CFD model with simplified treatment of radiative heat transfer is able to replicate the behavior of this type of receiver.
- CFD simulations campaigns â Results and discussion
Once validated, the CFD model was exploited to run a series of simulations campaign with the aim of evaluating how different operating conditions affect the Synhelion absorbing gas receiver. The most representative operating parameters evaluated were: (i) HTF mass flow rate, (ii) orientation of the HTF inlet velocity vector and (iii) gravity (upward- and downward-facing receiver). Moreover, two scenarios of receiver operating pressure, ambient pressure and 10 bars respectively, were also considered and compared. The beneficial effect of operating at higher pressure is the increased density of the HTF, which leads to a downsizing of the receiver proportional to the operating pressure increase. The reference receiver geometry assumed for the simulations, is 2D axisymmetric (figure 1b) with cavity dimensions of 16 m diameter and 16 m length, and 0.72 m diameter and 0.72 m length, in the case of unpressurized and pressurized receiver respectively. For both the cases, 600 kW/m2 concentrated solar flux on the absorptive surfaces was assumed with water vapor, the HTF under investigation, inlet temperature of 1000 K. The cavity is modelled as ideally insulated and therefore, the only source of heat loss is assumed to be through the aperture by means of thermal radiation only. The internal surfaces of the cavity are considered to have emissivity equal to 1 (black surfaces) and a fully diffusive emission of thermal radiation. Besides the thermo-fluid dynamics behavior, the overall receiver performance were also quantified by computing its thermal efficiency, defined as the ratio of the power removed by the HTF divided by the total input power.
Effect of HTF mass flow rate
This first CFD simulations campaign was devoted to evaluate the receiver performance operating with different HTF mass flow rate values. The reference HTF mass flow rate ranges considered were: 38 kg/s â 472 kg/s and 0.07 kg/s â 0.92 kg/s in the case of unpressurized and pressurized receiver respectively. The graph in figure 1c summarizes the resulting receiver thermal efficiency as a function of the HTF outflow temperature. The higher the HTF outflow temperature, the lower the HTF mass flow rate thorough the receiver. A consideration that can be drawn, is that reducing the HTF mass flow rate leads to a decrease of the receiver thermal efficiency due to higher heat losses. It is also interesting to observe the different evolution of the receiver performance with the efficiency that rapidly decreases moving away from the highest and the lowest HTF mass flow rate values considered. In between, the thermal efficiency curves show an inflection point in proximity of the HTF outflow temperature of about 1â500 K.
Effect of HTF entrance angle
Five different orientation of the HTF inlet velocity vector, as shown in figure 1b, were simulated in order to evaluate the effect of the HTF entrance angle on the receiver performance. The resulting receiver efficiency is reported in the graph of figure 1d. Based upon the obtained results, it can be concluded that inclination angles lower than about 30° from the aperture have a negligible effect on the receiver performance. An inclination angle of about 45° has a beneficial effect if the receiver operates at high HTF mass flow rates; conversely, it shows a detrimental effect in the case of lower HTF mass flow rate values. At higher inclination angles, the receiver efficiency seems to be generally higher; however, the receiver behavior is affected, at the same time, by the combined effect of HTF inclination angle and inlet velocity since the same inlet section was assumed for all the simulations.
Effect of gravity
In the case of large HTF density gradient, a gravity-induced buoyancy flow could take place affecting the flow field, and temperature distribution, into the cavity. Two evaluate the importance of gravity on the receiver performance, an additional CFD simulations campaign was performed assuming an upward- and downward-facing receiver. With these receiver orientations is possible to maintain the same 2D computational domain. The unpressurized receiver was assumed as reference. According to the results obtained, shown in figure 1e, the effect of gravity on the receiver performance is not negligible especially in the case of low HTF mass flow rates. As the HTF mass flow rate increases, the receiver performance are gradually less dependent on gravity up to a certain condition in which the effect of gravity can be safely neglected.
References
[1] C. Ho, Advances in central receivers for concentrating solar applicationsâ, Solar Energy 152, 38-56, (2017)
[2] G. Ambrosetti and P. Good, A novel approach to high temperature solar receivers with an absorbing gas as heat transfer fluid and reduced radiative losses. Solar Energy 183, 521-531 (2019).
[3] G. Ambrosetti, P. Good, P. Furler, L. Geissbühler, D. Rutz, S. Ackermann, Reaching Beyond 1â500°C With The Synhelion Absorbing Gas Solar Receiver: Results of Experimental Campaign, Abstract submitted for 2020 AIChE Annual Meeting.