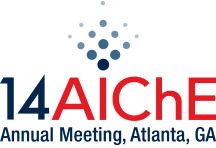
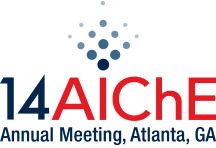
Steam Methane Reforming (SMR) is widely used for the mass production of synthesis gas and hydrogen. The heat required for the strongly endothermic SMR reactions is provided through the combustion of a fuel in a furnace in which the packed bed SMR reactor tubes are suspended. The CO2 produced in the furnace is mixed with N2 and, hence, challenging to capture. To respond to growing concerns on CO2 emissions and the energy cost of SMR, autothermal Chemical Looping Reforming technology (a-CLR) has been recently developed [1-3]. A circulating fluidized bed system is proposed. The catalyst serves for the transport of heat and oxygen between the catalyst re-oxidation reactor and the SMR reactor. In the latter, the catalyst is reduced and heat is net consumed. Direct contact between fuel and air is avoided. Because of the use of fluidized bed technology, operation at low pressure is preferred and smaller catalyst particles can be used. This allows avoiding the strong intra-particle diffusion limitations faced in the conventional packed bed SMR reactors.
Studies on a-CLR focus on lab- and pilot-scale demonstration [4-10] and on the influence of different operating variables [11]. The reaction mechanism is typically described in terms of global reactions only. A key issue for the scale-up of a-CLR technology is the development of a simulation model that accounts for the details of the reaction mechanism and kinetics. The modeling and simulation of a-CLR on a Ni-MgAl2O4 catalyst is addressed in this work. Based on kinetic modeling studies on methane steam reforming [12-19], the partial oxidation of methane [20-22], and the re-oxidation of the catalyst [23], a detailed reaction mechanism is constructed and the reaction kinetics derived. The rate equations account for the balance between oxidized and non-oxidized metal catalyst sites, oxidized sites not being active for the steam reforming and water gas shift reactions. Different reaction steps for the reduction of the metal oxide by hydrogen or methane are considered. A heterogeneous reactor model is used and validated by means of lab- and pilot scale data from the literature [9,10]. Next, commercial scale a-CLR is considered and the influence of the operating conditions studied. The conversions that can be achieved and the eventual overheating of the catalyst and related risk of sintering during the catalyst re-oxidation are evaluated.
References:
[1] Ortiz M, Abad A, de Diego LF, Garcia-Labiano F, Gayan P, Adanez J, Int. J. Hydrogen Energ., 36, 9663-9672, 2011.
[2] Moghtaderi B, Energy Fuels, 26, 15-40, 2012.
[3] Adanez J, Abad A, Garcia-Labiano F, Gayan P, de Diego LF, Prog. Energ. Combust., 38, 215-282, 2012.
[4] Ryden M, Lyngfelt A, Mattisson T, Fuel, 85, 1631-1641, 2006.
[5] Ryden M, Lyngfelt A, Mattisson T, Energy & Fuels, 22, 2585-2597, 2008.
[6] Ryden M, Johansson M, Lyngfelt A, Mattisson T, Energy Environ. Sci., 2, 970-981, 2009.
[7] de Diego LF, Ortiz M, Garcia-Labiano F, Adanez J, Abad A, Gayan P, J. Power Sources, 192, 27-34, 2009.
[8] Pröll T, Bolhàr-Nordenkampf J, Kolbitsch P, Hofbauer H, Fuel, 89, 1249-1256, 2010.
[9] Linderholm C, Mattisson T, Lyngfelt A, Fuel, 88, 2083-2096, 2009.
[10] Kronberger B, Lyngfelt A, Löffler G, Hofbauer H, Ind. Eng. Chem. Res., 44, 546-556, 2005.
[11] Ortiz M, de Diego LF, Abad A, Garcia-Labiano F, Gayan P, Adanez J, Int. J. Hydrogen Energ., 35, 151-160, 2010.
[12] Xu J, Froment GF, AIChE J., 35, 88-96, 1989.
[13] Snoeck J-W, Froment GF, Ind. Eng. Chem. Res., 41, 3548-3556, 2002.
[14] Snoeck J-W, Froment GF, Ind. Eng. Chem. Res., 41, 4252-4265, 2002.
[15] Wei J, Iglesia E, J. Catal., 224, 370-383, 2004.
[16] Wei J, Iglesia E, J. Catal., 225, 116-127, 2004.
[17] Wei J, Iglesia E, J. Phys. Chem. B, 108, 4094-4103, 2004.
[18] Jones G, Jakobsen JG, Shim SS, Kleis J, Andersson MP, Rossmeisl J, Abild-Pedersen F, Bligaard T, Helveg S, Hinnemann B, Rostrup-Nielsen JR, Chorkendorff I, Shested J, Norskov JK, J. Catal., 259, 147-160, 2008.
[19] Blaylock DW, Ogura T, Green WH, Beran GJO, J. Phys. Chem. C, 113, 4898-4908, 2009.
[20] De Groote AM, Froment GF, Appl. Catal. A: General, 138, 245-264, 1996.
[21] Slaa JC, Berger RJ, Marin GB, Catal. Let., 43, 63-70, 1997.
[22] Trim DL, Lam C-W, Chem. Eng. Sci., 35, 1405-1413, 1980.
[23] Hatcher WJ, Viville L, Froment GF, Ind. Eng. Chem. Process Des. Dev., 17, 491, 1978.