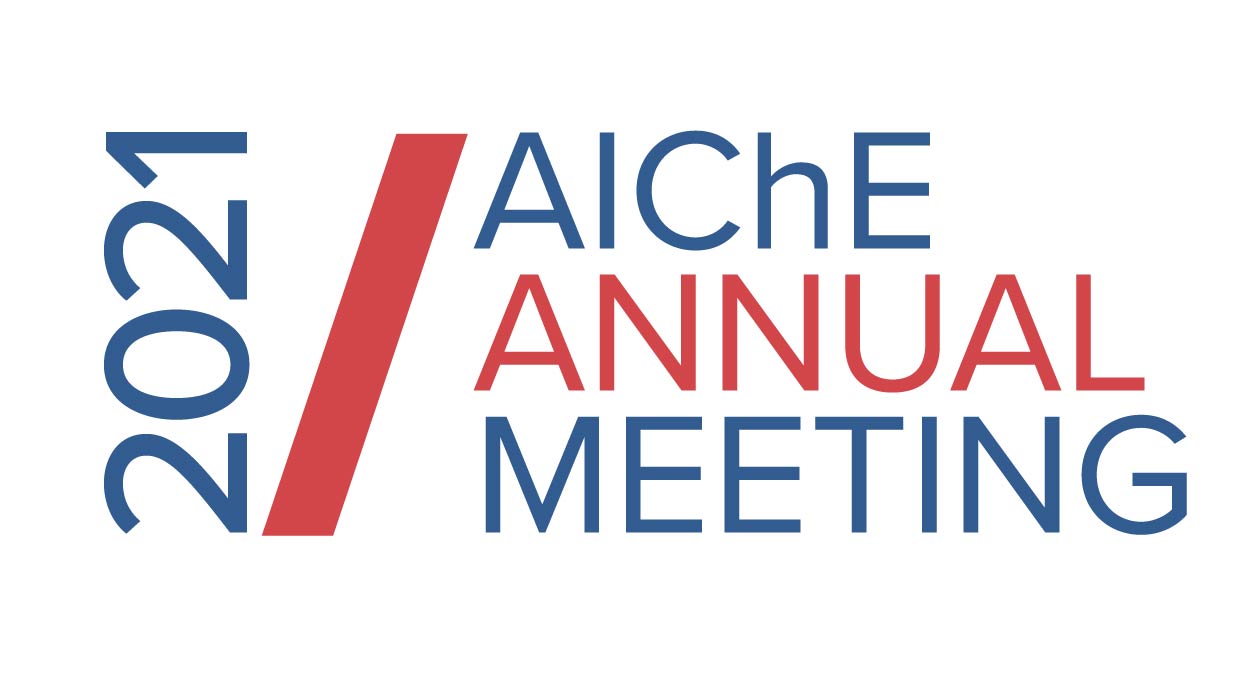
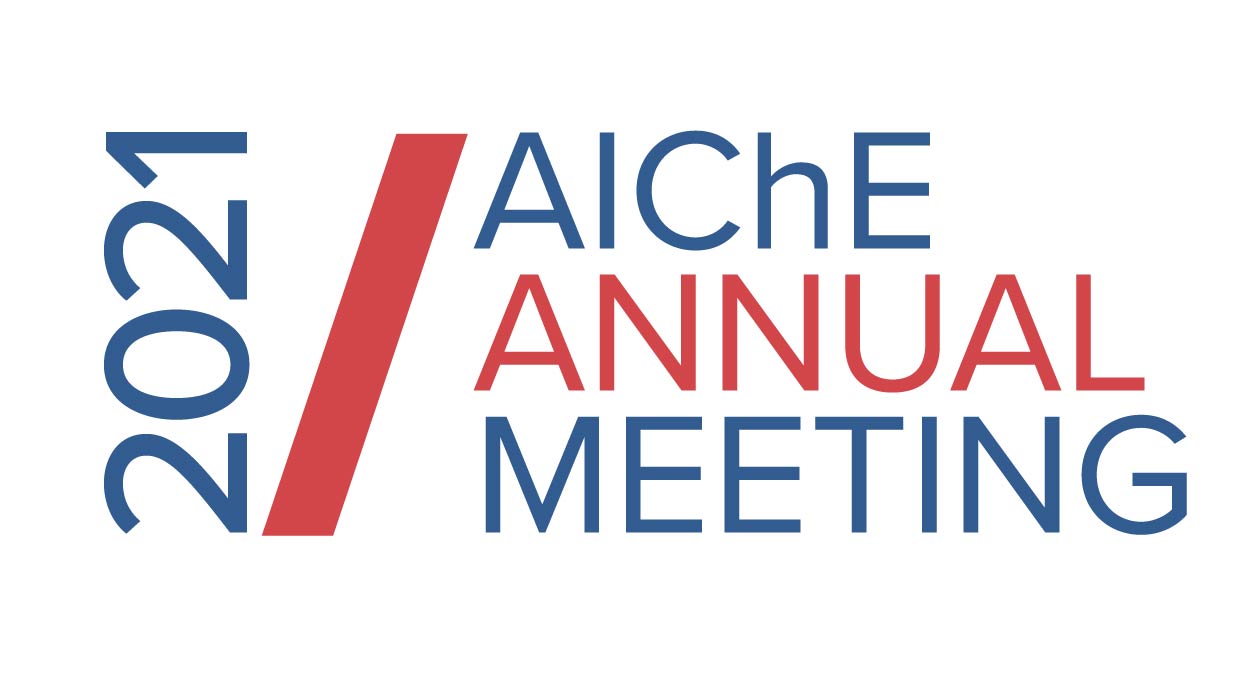
These goals require large-scale nuclear biorefineries (250,000 barrel per day equivalent) that can economically produce a variable product slate (hydrocarbon fuels, hydrocarbon chemical feedstocks and sequestered carbon dioxide) based on market prices. If biomass feedstock costs or liquid fuel prices are low in a particular year, more pure carbon dioxide will be produced for sequestration assuming there is a market for negative emissions. If hydrocarbon fuel prices are high, there may be no carbon dioxide sequestered in a particular year. The market (including price of sequestered carbon dioxide) determines the relative quantities of different products. The core of the refinery is similar or identical to existing large integrated oil refineries with two major changes.
Front end processing. The front end converts biomass into a synthetic crude oil. The baseline process is Fischer Tropsch (gasifier, water shift reactor and FT synthesis) to produce the hydrocarbon âcrude oilâ for the refinery. The Fischer Tropsch process is used today to convert coal and natural gas into liquid hydrocarbon fuels at a commercial scale. Varying heat and hydrogen input to the gasifier and water-shift reactor shifts relative production of hydrocarbons and carbon dioxide. There are alternative processes to convert different types of biomass into synthetic crude oil for the refinery.
Nuclear heat and hydrogen. Nuclear energy is used to provide heat and hydrogen for all refinery operations. Nuclear heat and hydrogen input at the refinery is the enabling technology for sufficient biomass feedstocks to meet liquid fuel demands without major impacts on agriculture and forestry, including food and fiber costs and availability. This objective is achieved by two mechanisms. Biomass has a typical composition near CH1.44O0.66 that must be converted to a hydrocarbon fuel with a composition near CH2. The oxygen can be removed by using carbon in the biomass to create carbon dioxide. We can remove more carbon to obtain the proper carbon to hydrogen ratio and provide energy for the process. Alternatively we can add external heat and hydrogen to operate the process and remove the oxygen as water. This requires massive amounts of heat and hydrogenâbut this approach can double liquid hydrocarbon fuels per unit of biomass feedstock. The only low-carbon sources of concentrated heat and hydrogen at the required scale are nuclear and fossil fuels with carbon capture and storage (CCS). The second consideration is that there are many sources of biomass that are good carbon sources but relatively poor energy sources (double-cropped biomass, sewage sludge, garbage, etc.). These are viable low-carbon feedstocks if external heat and hydrogen inputs to the biorefinery are provided by nuclear plants.
The required large-scale nuclear biorefineries require viable systems to deliver biomass to the refinery front gate [Fig. 1]. Large nuclear-powered biorefineries require massive quantities of feedstocks, on the order of 30,000 tons per day. There is insufficient low-density biomass within an economic transport distance to meet the feedstock demand of such large biorefineries. It is prohibitively expensive to ship low-density biomass long distances to such large refineries. What is required are intermediate processing facilities called âdepotsâ to consolidate near the farm or forest the local biomass into stable high-density forms that can be economically shipped long distances to large-scale nuclear bio-refineries. This is equivalent to shipping dense storable #2 corn to ethanol plants. As a point of comparison, the largest corn milling plant receives about 15,000 tons of corn per day by unit train.
There are multiple potential depot processes to create a storable high-density intermediate product: pelletization, pyrolysis, and anaerobic digestionâeach sending different intermediate high-density intermediate products to the nuclear bio-refinery with different secondary products. These secondary âwastesâ include biochar and anaerobic digester waste that are fertilizers to recycle stable carbon, phosphorus and other nutrients back to the land. Biochar can be used to build up soil and sequester carbon or as a feedstock for the refineryâdepending upon market incentives for negative carbon emissions.
Depot processes are directly coupled to agricultural processes to assure long-term sustainability. The storable shippable product creates a biomass refinery feedstock market similar to that for corn or, on much smaller scale, wood chips. The system fits within the existing American agricultural system and creates a new market for agriculture. Since the 1930s, the primary challenge of American agriculture has been excess production capacity. The excess production capacity now has a very large potential marketâproviding energy services and low carbon feedstocks for petrochemical production.
The full potential of this system to produce biomass is not well understood. Historically, biomass studies have examined the availability of biomass as an energy source. Such studies indicate that biomass could provide up to a quarter of global energy demand. The question here is different: What is the potential to provide enough carbon from biomass to replace all liquid fuels, hydrocarbon feedstocks to the chemical industry and negative carbon emissionsâwhere we have massive hydrogen and heat input from nuclear sources to convert that biomass into useful products? Total global biomass produced annually by photosynthesis is about 100 billion tons; thus, the total resource base is sufficient. The question is price. This question has two subcomponents. What could American agriculture produce if there was a market? What is the carbon biomass from feedstocks that are not good fuels but excellent carbon sources? Preliminary studies indicate sufficient feedstocks to meet world liquid fuel demandâprovided nuclear heat and hydrogen inputs at the refinery so the biomass is first considered as a carbon feedstock and secondarily as an energy source. The at-the-farm or forest biomass prices per ton today are similar to crude oil prices.
We have started to undertake studies and workshops to map out the system options with base-line and alternative optionsâand the limits to each option. Where are the bottlenecks? What are the primary economic drivers? Some parts of this system are well-understood; eg, large-scale industrial technologies such as integrated refineries. Some parts of this system such as Fisher Tropsch are existing technologies used in some markets but not for this specific market at this scale. Other components such as depot processes have been demonstrated at pilot scale.
The other requirement is to understand the economics and market drivers. This includes the potentially future market for negative carbon emissions. The expectation is that ultimately there will be a tax on carbon dioxide emissions. If there is a tax, there should logically be a market for negative carbon emissions; that is, removing carbon dioxide from the atmosphere and sequestering carbon dioxide. The economics of carbon capture with fossil fuel power plants is that carbon capture is expensive (carbon dioxide diluted with air) but carbon sequestration is relatively cheap if a reasonably pure stream of carbon dioxide is sequestered. Refinery biomass gasifiers and the water gas shift reaction to provide syngas (a mixture of hydrogen and carbon dioxide) to the Fischer Tropsch process can be operated to provide various ratios of syngas and carbon dioxideâbased on heat and hydrogen inputs. If there is a large market for negative emissions, it provides the refinery two revenue streamsâfuels and negative carbon emissions where variable output is based on biomass feedstock costs and the prices for the two products. Negative carbon emissions become the variable product that in some years would be a major product but in other years perhaps little or no production. From a climate perspective, what is important is negative emissions over decadesânot over a single year.
[1] C. Forsberg and B. Dale, âReplacing Liquid Fossil Fuels with Liquid Biofuels from Large-Scale Nuclear Biorefineriesâ Volume 8; Proceedings of Applied Energy Symposium: MIT A+B, United States 2020, August 12-14, 2020, Cambridge, MA. https://applied-energy.org/mitab2020/program
[2]. C. Forsberg et al., Workshop: Can a Nuclear Biofuels System Enable Liquid Biofuels as the Economic Low-carbon Replacement for all Liquid Fossil Fuels and Chemical Industry Inputs, Webinar (August 4, 11, 18), Massachusetts Institute of Technology, Michigan State University, North Carolina State University. (Proceedings in preparation)