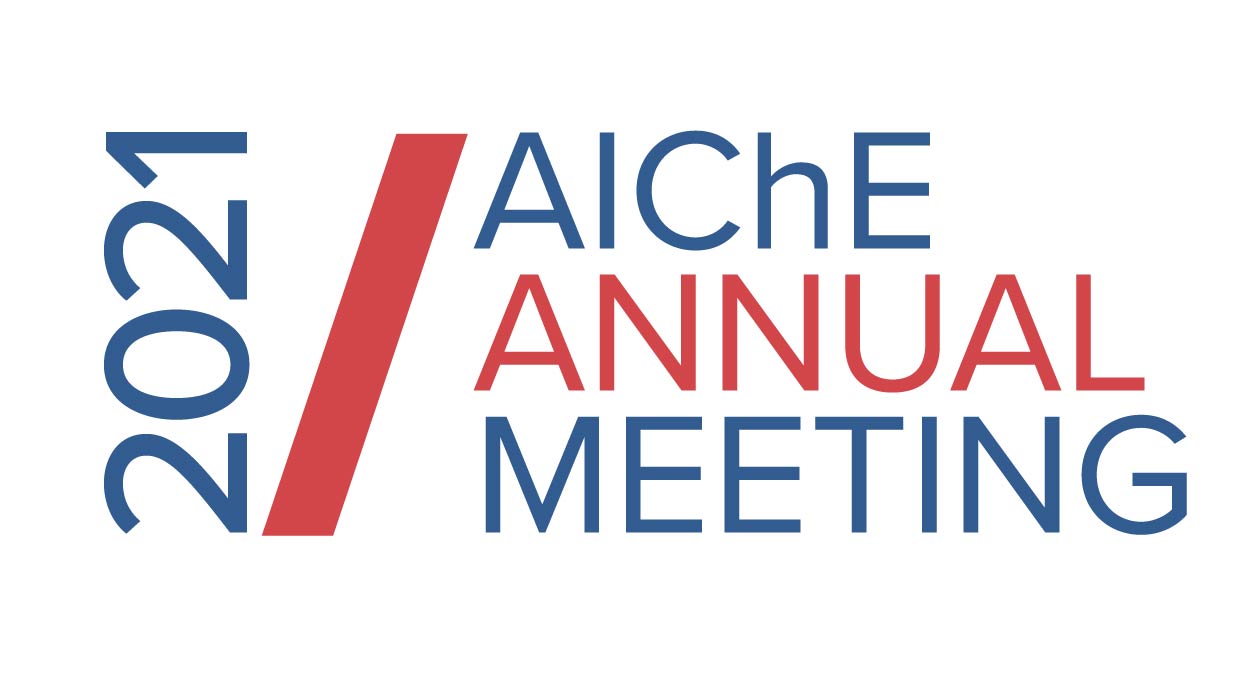
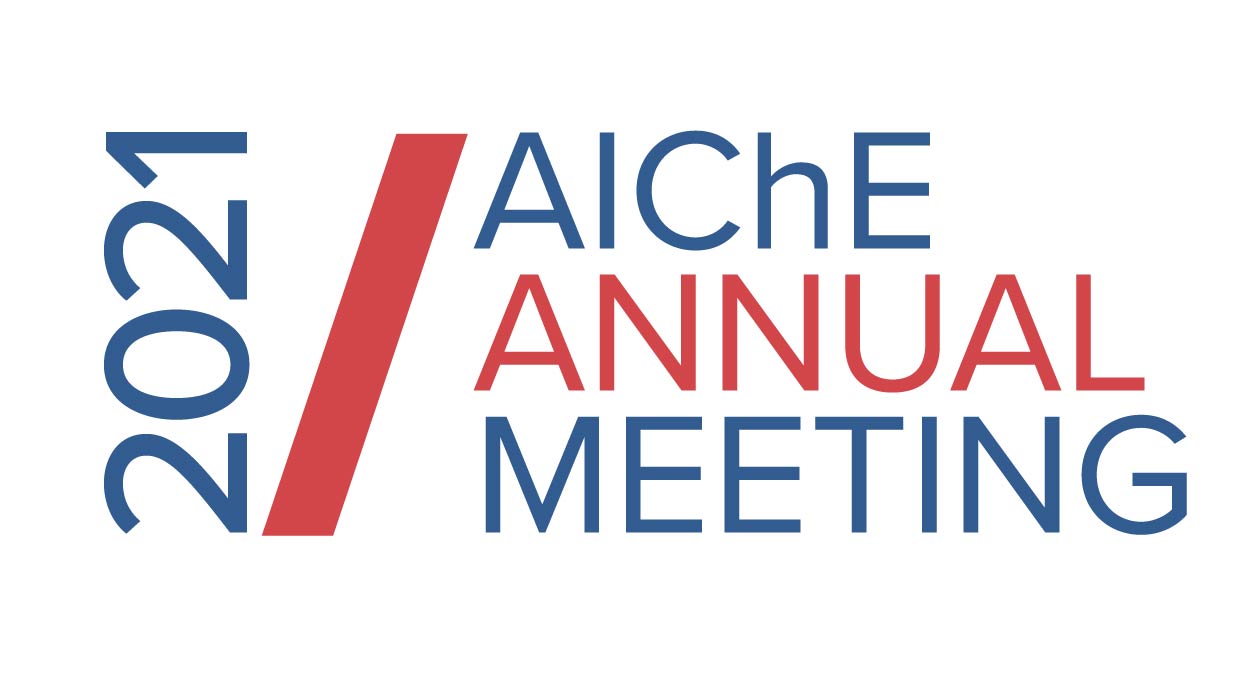
While optimism grows with the introduction of COVID-19 vaccines, widespread mass testing—up to 14 million tests per day (1) in the United States alone—will be needed for the foreseeable future. Sample pooling, an efficient method to test large numbers of people, saves on healthcare resources, improves turn-around times on results, and ultimately could enable the high testing capacity necessary to meet this demand (2). However, sample pooling using the gold standard for SARS-CoV-2 detection, RT-qPCR, is inherently limited: because its sensitivity decreases as sample dilution increases, RT-qPCR’s false negative rate increases with sample pool size (2). Additionally, RT-qPCR is susceptible to amplifying residual fragments of the viral genome, even in the absence of infectious virus (3)(4). Thus, there is an urgent need for novel assays that retain high sensitivity when samples are diluted and that detect only intact virus, which is better correlated with infectivity than viral RNA (3).
We have developed an innovative, highly sensitive method, Detection of Intact Virus by Exogenous-oligonucleotide Reaction (DIVER), to detect and quantify SARS-CoV-2 in pooled samples for community surveillance. DIVER is based on: 1) labeling viral particles with exogenous single-stranded DNA oligonucleotides (oligos); 2) capturing the labeled viral particles with paramagnetic beads coated with ACE2, the primary receptor on human cells to which SARS-CoV-2 binds; and 3) performing qPCR on the oligo labels, which produces a signal that increases linearly with viral particle count (Fig. 1A). We utilized two models to demonstrate DIVER safely under Biosafety Level 2 conditions: liposomes displaying the SARS-CoV-2 spike protein (5) and a Spike-pseudotyped lentivirus. With these models, we show that DIVER’s sensitivity is relatively unaffected by sample dilution and, as such, DIVER is uniquely suited for sample pooling.
EXPERIMENTAL
To detect liposomes or lentivirus from dilute solutions, particles were tagged with exogenous oligos. Specifically, we prehybridize a cholesterol-linked anchor oligo (length = 46 nucleotides (nt), IDT) to a detection oligo (length = 130 nt, IDT). Upon addition of the resulting complex to the particles suspended in phosphate buffered saline (PBS) solution, the cholesterol molecules intercalated nonspecifically into the lipid-bilayer membrane, tagging the particles with oligos. To stabilize the cholesterol-oligos in the membrane, we introduce a cholesterol-linked co-anchor oligo (length = 20 nt, IDT) that also intercalates in the membrane, allowing the co-anchor to hybridize with a complementary region of the primary anchor (Fig. 1B). Particles were then captured using paramagnetic beads (Dynabeads M-270 Streptavidin, Invitrogen) functionalized with biotinylated ACE2 (Acro Biosystems). Beads were washed five times to remove free oligo and off-target particles. Exogenous oligo tags were then quantified with qPCR.
RESULTS AND DISCUSSION
We first demonstrated that the DIVER output signal – normalized qPCR threshold cycle – is correlated with the number of S-liposomes. Using a dilution series (1x104-1x107 particles in 10 µL), we evaluated our ability to discern S-liposomes from liposomes without surface protein (“plainâ€). We considered the difference in threshold cycle (dCT) between each sample and a no-liposome control containing all other assay components processed in parallel to ensure that we quantified only labels associated with liposomes. Samples with at least 1x105 S-liposomes had significantly higher dCTs than those containing just 1x107 plain liposomes (1x105, p < 0.01; ≥ 1x106, p < 0.0001).
To mimic extracellular vesicles abundant in biofluids, we mixed CD63-liposomes with S-liposomes. Analyzing a dilution series of S-liposomes in a background of 1x108 CD63-liposomes, we measured a significant difference in dCT between samples containing at least 1x106 S-liposomes and just 1x108 CD63-liposomes or 1x108 plain liposomes (p < 0.01 and p < 0.0001, respectively; Fig. 1C), suggesting that our method retains high sensitivity even in the presence of biologically relevant numbers of off-target lipid bilayer nanoparticles.
We next validated DIVER’s ability to detect low numbers of intact viral particles displaying spike protein in a heterogenous mixture. We analyzed 100 plaque-forming units (pfu) of Spike-pseudotyped lentivirus or VSV-G-pseudotyped lentivirus and an equivalent volume of no-virus control media, captured on either ACE2 or anti-VSV-G (aVSV-G) beads. We observed a significantly larger signal for Spike-pseudotyped lentivirus than for VSV-G-pseudotyped lentivirus incubated with ACE2 beads (p < 0.01; Fig. 1D). Using RT-qPCR to determine lentivirus infectivity coefficients, we established that DIVER is able to detect robustly viral particles displaying spike protein corresponding to an RNA copy number of less than 1x104.
Finally, we demonstrated our method’s sample pooling capability by performing two different blinded experiments. We used three rounds of pooling to determine the positive sample, representing a 42% reduction in the number of tests required compared to processing each sample individually. In the first pooling experiment, 12 mock samples contained 1x107 CD63-liposomes/µL, and one sample also contained 1x106 S-liposomes/µL. 10 µL of each sample was processed for each round of pooling. Using a threshold of 0.5 cycles, DIVER correctly identified the positive pool or sample for each round. For confirmation, all samples were then processed individually; the dCT for negative pools or individual samples was 0.032 ± 0.273 (mean ± standard deviation), emphasizing our method’s negligible background signal for lipid bilayer nanoparticles not displaying spike protein.
In the second pooling experiment, we identified one positive sample from a large pool of samples with biologically relevant virus:EV ratios. Here, 12 mock samples contained 4.02x106/µL cell-culture derived EVs, and one sample also contained 20 pfu/µL Spike-pseudotyped lentivirus. As before, 10 µL of each sample was processed for each round of pooling, and using a threshold of 0.6 cycles, we correctly identified the positive pool or individual sample for each round.
CONCLUSION
DIVER addresses several of the shortcomings of RT-qPCR. It detects only intact virus, which is more indicative of infectiousness than viral RNA (3), and it is better suited for sample pooling, as it is more robust to sample dilution. In addition, DIVER does not require RNA extraction or reverse transcription, eliminating the need for reagents that have faced supply-chain challenges globally. DIVER’s greater sensitivity to the absolute number, rather than the concentration, of viral particles in a sample is critical to the detection of SARS-CoV-2 in inherently dilute samples such as the pooled biosamples (6) and wastewater (7) that are currently screened for community surveillance. Furthermore, DIVER is readily adaptable to any enveloped virus simply by changing the capture moeity, enabling its rapid deployment should a novel enveloped viral pathogen emerge in the future. By enabling larger sample pools while reducing the risk of false negatives, DIVER is uniquely positioned to contribute to the expanded community surveillance necessary to suppress COVID-19 and future pandemics.
REFERENCES
1. C. Silcox et al., "A National Decision Point: Effective Testing and Screening for Covid-19," , 2020).
2. B. Cleary et al., Using viral load and epidemic dynamics to optimize pooled testing in resource-constrained settings. Science Translational Medicine., eabf1568 (2021).
3. C. Huang et al., Culture-Based Virus Isolation To Evaluate Potential Infectivity of Clinical Specimens Tested for COVID-19. J. Clin. Microbiol. 58, 270-8 (2020).
4. J. Lu et al., Clinical, immunological and virological characterization of COVID-19 patients that test re-positive for SARS-CoV-2 by RT-PCR. EBioMedicine. 44, 50-59 (2019).
5. M. Kozminsky, T. R. Carey, L. L. Sohn, DNA-directed patterning for versatile validation and characterization of a lipid-based nanoparticle model of SARS-CoV-2. ChemRxiv.(2021).
6. C. A. Hogan, M. K. Sahoo, B. A. Pinsky, Sample Pooling as a Strategy to Detect Community Transmission of SARS-CoV-2. Jama. 323, 1967-1969 (2020).
7. W. Randazzo et al., SARS-CoV-2 RNA in wastewater anticipated COVID-19 occurrence in a low prevalence area. Water Research. 181, 115942 (2020).
8. M. E. Todhunter et al., Fabrication of 3D Reconstituted Organoid Arrays by DNA-programmed Assembly of Cells (DPAC). Curr Protoc Chem Biol. 8, 147-178 (2016).