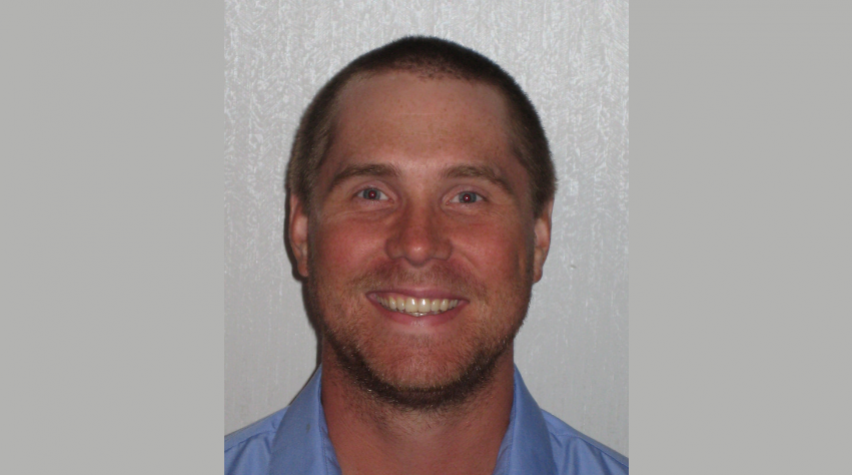
As part of AIChE's 110th Year Celebration, this series provides perspectives on the future of chemical engineering from dozens of leaders in industry, academia, and at national laboratories.
We continue with Marc Birtwistle, Associate Professor of Chemical Engineering at Clemson University. In 2008, he was completing his PhD research at the University of Delaware with Prof. Babatunde Ogunnaike.
During AIChE’s centennial year of 2008, AIChE interviewed Dr. Birtwistle to learn his perspectives on the future of chemical engineering. In today’s blog post, we compare some of Birtwistle’s comments from 2008 with his perspectives today.
A chemical engineer’s traditional role in the pharmaceutical industry is in formulation, scale-up, and/or production, all primarily post-drug development. However, the industry is changing. The drug development pipeline is reaching a tipping point, being squeezed by ever-increasing costs coupled with declining success rates.
In 2008, Birtwistle shared these thoughts about opportunities in chemical engineering:
Incorporation of systems biology into chemical engineering as a core science can have significant impact on multiple industry sectors by enabling rational engineering and manipulation of biological systems ranging from bacteria to humans. Systems biology is an emerging discipline focused on understanding how the behavior of living systems arises from their individual pieces.
In the petrochemical sector, series of energy-intensive reactors and separators can be replaced with a single vessel containing rationally engineered microorganisms capable of performing multiple catalytic steps. Additionally, customized biomass feedstocks with optimal processing properties can help to replace dwindling oil feedstocks. The net effect of increased use of renewable raw materials, coupled with decreased energy consumption, will be a more sustainable petrochemical industry.
The pharmaceutical and biomedical sectors can be impacted by understanding of human systems derived through systems biology. “Network-based drug design” using predictive models for how drug-induced changes at the molecular level affect physiological function, would allow the pharmaceutical sector to become more cost-effective by abandoning potentially ineffective or dangerous drug candidates early rather than late in the drug-development pipeline.
The biomedical sector can use such predictive models for “model-based personalized medicine,” to customize multivariate treatments for diseases such as cancer or autism that arise from non-unique interactions between numerous environmental and genetic factors.
As systems biology matures over the next 25 years, in addition to these impacts on existing sectors, we may also see the arrival of a new sector devoted to modeling, design, production, and utilization of engineered living systems.
In a new 2018 interview, here's what Birtwistle said:
Looking 25 years into the future, how do you expect your industry/research area to evolve?
I expect computational models based on chemical engineering principles to play larger roles during drug development. Such models may allow better prediction for how complex multifactorial diseases such as cancer will respond to drugs and drug combinations, or how otherwise healthy organ systems may have adverse reactions to the same.
This can help the pharmaceutical industry cull potentially dangerous drug candidates earlier in the pipeline, prior to costly late stage work; better match drugs to patients more likely to respond to therapy; avoiding failed clinical trials due to poor inclusion or exclusion criteria; and rationalize choices for drug combination therapy, which quickly becomes intractable to explore experimentally but is more attainable through simulation.
This application of systems biology is often termed systems pharmacology. However, much basic research is still needed to understand how current modeling methods can be meaningfully applied to very complex, multi-scale, and incompletely understood biological systems.
Core areas of ChE expertise are being augmented by new expertise in science and engineering at molecular and nanometer scales, in biosystems, in sustainability, and in cyber tools. Over the next 25 years, how will these changes affect your industry/research area?
Technological advances are progressing faster than ever in biomedical science; a prototypical example is DNA sequencing, where the cost of sequencing a human genome decreased from ~$100 million to $1,000 in 15 years.
Such advances are making ever larger amounts of data not only obtainable, but also accessible through renewed and increased efforts to archive, annotate, and sustain such data in publicly available resources, such as The Cancer Genome Atlas or the Cancer Cell Line Encyclopedia. When coupled with similar fast-paced increases in computing power, opportunities arise to leverage these advances toward systems pharmacology efforts.
Statistical data mining and other big data approaches alone are, in my opinion, unlikely to be sufficient in such regards; first-principles and mechanism-based thinking that defines us as chemical engineers will almost certainly be required to help separate signal from noise in the march toward improved ability to simulate and predict human system responses to therapy.
What new industries/research areas do you foresee?
I see chemical engineers becoming more involved with clinical health care decision making. I use cancer here as an example, but the reach is not limited to this. Currently, cancer precision medicine is based on matching genomic data to targeted drugs. This has revolutionized cancer care, but is not always successful. Usually, this matching is a one-mutation-to-one drug, but that ignores the reality of complex, dynamic, heterogeneous, and adaptive biochemical systems lying between genetic aberrations and drug action.
Just as simulation models could help the pharmaceutical industry, one could envision that the same such models could help inform patient care. Patient-specific measurements can parameterize simulation models that then prioritize likely efficacious drugs or drug combinations.
Systems pharmacology models could also account for important features of drug action, such as on- and off-target effects, potential toxicity, dosing, dynamics, and sequence. In parallel, chemical engineers are trailblazing nanomedicine technologies for delivery of drugs in new ways. Such abilities are synergistic with simulation models that try to capture drug response behavior, which can allow an exploration of which delivery mechanisms are likely to be more effective.
Taking into account the ongoing evolution of the professions — including the need for new modes of education; high standards of performance and conduct; effective technical, business, and public communication; and desires for a more sustainable future — what do you think the chemical engineering profession will look like 25 years from now?
There will always be a role for chemical engineers in traditional applications such as process engineering, regardless of how raw material inputs and desired products evolve. Core training in kinetics, thermodynamics, separations, transport, and control will similarly remain valuable. These skills, when combined with the problem-solving abilities that our curriculum infuses, produce a versatile individual that thrives both in traditional roles and in pioneering new fields, such as cancer systems biology and pharmacology, synthetic biology, or drug delivery.
However, the tools and skills required to convert this training into actionable and desirable abilities will need to evolve with current technology. Big data and its analysis —which includes branches of computer science such as machine learning, classification, and programming — still appear somewhat lacking from a typical chemical engineer’s toolbox. Yet, these skills are becoming increasingly important and I expect their application to be ubiquitously powerful, albeit not a cure-all by themselves.
Likewise, continued permeation of biology as a core science into chemical engineering curricula will help our profession adapt to changing landscapes increasingly focused on sustainability and health care. The acceptance of biology as a core chemical engineering science on the same level as chemistry and physics has not yet been achieved in my view and needs to accelerate.
On a different note, historically, chemical engineers have focused on scale-up. However, it would seem that smaller processes have many advantages, including their environmental impact, ability to adapt to changing markets, lower capital requirements, and better safety profiles, to name a few. Thus, we may see a shift from focus on scale-up to scale-down — requiring expertise in so-called process intensification. I would expect advances in engineered biological systems to play a large role in such scale-down.
AIChE's 110 Year Celebration
Celebrate AIChE's 110-year anniversary. Attend this Annual Meeting session, focusing on the future of chemical engineering through the eyes of thought leaders from industry, academia, and national laboratories.