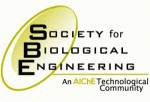
By: Sarah Widder
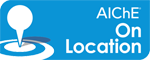
This session presented work from a number of groups from one of three Bioenergy Research Centers sponsored by the DOE; the Great Lakes Bioenergy Research Center (GLBRC), BioEnergy Science Center (BESC), and Joint BioEnergy Institute (JBEI). A major focus of the Bioenergy Research Centers is to understand how to reengineer biological processes to develop new, more efficient methods for converting the cellulose in plant material into ethanol or other biofuels that serve as a substitute for gasoline. This session focused on the characterization and breakdown of lignin, a main component of plant cell walls.
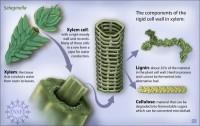
John Sedbrook of Illinois State University
Sedbrook, the first speaker, whose research came from GLBRC in collaboration with UW Madison and Michigan State, explained how plant cell walls are rich in polysaccharides. The goal, Sedbrook said, is to break down the cell wall to get to the long glucose chains. However, plant cell walls are extremely complex with lots of different kinds of polysaccharides with complex linkages, all contained within a lignin cover. This lignin is an essential component to provide stability and strength, assist in water transport, prevent against dehydration, and protect plant from pathogens. However, lignin is difficult to breakdown. It requires alkalide environments and high temp or acid to break it down. And, when you do break it down, lignin generates fermentation inhibitors. Therefore, this typically represents the hardest part in utilizing lignocellulosic feedstocks for bioenergy.
Sedbrook's research is trying to increase the abundance and accessibility of hemi-cellulose. Sedbrook is attempting to do this by developing a "designer" lignin with a biosynthesis pathway that has been altered using tranformase enzymes that form ester conjugates. One type (FMT) can "zip" or be incorporated into lignin structure and creates a linkage between the FMT and the lignin is more susceptible to alkaline. This makes the lignin easier to break down at lower temperatures.
Another approach is to regulate lignin in secondary cell walls. This is accomplished by regulating the RLX 1 gene. The group found that plants grown with less RLX1 gene grew less lignin, which caused the plants to have weaker stems so they fell over. The team verified that this was the case by using different lignin assay and staining techniques showed less lignin grown, and more hemi-cellulose.
David Mann of the University of Tennessee, in the BESC group
The next talk by Mann, discussed advances in switchgrass biotechnology to achieve improved feedstocks for lignocellulosic biomass conversion. Mann began by presenting why switchgrass is such a good candidate for bioenergy uses, not least of which is the measured 5:1 energy ratio and touted pest resilience. Mann also introduced a term for the problems, "biomass recalcitrance." Mann explained is as the plants lack of cooperation in being broken down." The first step in improving plant "cooperation," Mann said, is understanding how plants are biosynthesized. Mann discussed how by reducing the lignin levels, altering the type S lignin to the type G lignin ratios, and homogenizing lignin, much can be done to achieve better conversion from biomass to sugar.
-
- Switchgrass provides many opportunities for bioenergy
Dr. Pu from BSEC
The third speaker moved from switch grass to poplar, discussing the characterization of poplar lignin with and without dilute acid pretreatment. Dr. Pu took poplar, which was willey milled using 60-80 mesh before being pretreated with sulfuric acid at 170?C with 0.5 wt % sulfuric acid. After pretreatment, the slurry was cooled and vacuum dried. The dried lignin compounds were isolated with benzene ethanol extraction, ball milling, and dioxane extraction. Dr. Pu was able to characterize the remaining residue was characterized using C13 NMR.
The fourth speaker, from NREL, discussed the bioengineering of lignin in alfalfa. Specifically, this research found that
increasing the H type lignin, which have no methoxy- groups and thus are not as reactive, produced biomass that was more extractable. The NREL group achieved increased H type lignin by downregulating C3H and HCT. These engineering plants exhibited lignin extraction at 110?C with 2M basic solution for 10 minutes. With a typical plant, this would not cause any lignin extraction to occur. The group hypothesizes that this increased extractability is caused because the H lignin is smaller, even though there are more carbon bonds, and thus is easier to break down and extract.
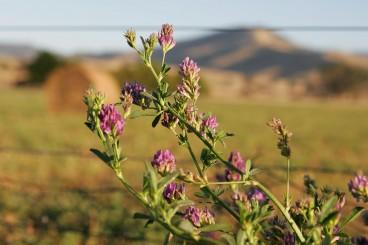
The fifth speaker discussed useful NMR techniques to characterize biomass in addressing the issue of recalcitrance. The speaker presented six methods:
- Solution NMR with a 2D correlation spectrum, which achieves higher resolution to better differentiate peaks;
- Ionic Liquid NMR which can be used instead of Classical Lignin Extraction. This means you can analyze the sample in solution and resolve the signals without breaking down cell walls;
- Use of a phosphilating agent to isolate important parts of lignin;
- Solid state NMR, which can be used to see crystallization. However, the speaker has found that crystallization may not be as important as some other characteristics in terms of biomass recalcitrance;
- NMR relaxation, which can look at pore size in cell walls; and
- C13 selective excitation and spin diffusion NMR. This is accomplished by magnetizing a certain region and observing how fast it spreads to other regions. This gives you information about how far away the lignin is from cellulose and can help characterize the spatial distribution of the cell wall.
The last speaker, from JBEI discussed the development of a feedstock agnostic pretreatment approach for bioenergy crops. The method they developed is to add ionic liquid to biomass and heat it up to 160?C. Water is then added to cause the solubalized cellulose to precipitate out. The biomass solubalizes because hydrogen-hydrogen bonding between the ionic liquid and biomass is greater than the bonding within the biomass. The team found that as temperature increases, they could achieve higher lignin solubalization and extraction, as would be expected. While there were some differences between different types of biomass (trees vs. grasses, etc) at lower temperature, at higher temperature (160C) all biomass reacted similarly. Further analysis proved that ionic liquid extraction performed better than acid pretreatment. While ionic liquids can be expensive, the research team also found that the ionic liquids used can be recycled with no decrease in efficiency, which makes this process cost comparative with other methods.
It is the author's opinion that if there potential for decreasing the cost of ionic liquids, then this would seem very attractive.