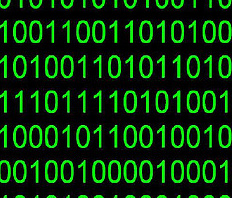
In an era where computer modeling has revolutionized various engineering fields, biologists have remained relatively untouched by the trend until now. But Stanford researchers announced late last week in an article published in the journal Cell that they had completed the first computer model for an entire organism.
The researchers, led by Markus Covert, assistant professor of bioengineering, drew data from more than 900 scientific papers to account for every molecular interaction that takes place in the life cycle of Mycoplasma genitalium, the world's smallest free-living bacterium. By creating a complete model, the team has ushered in a new era in biology and biological engineering where researchers will be able to eliminate a significant amount of time-consuming and expensive trial and error by manipulating a computer model before moving to real biological specimens.
Why Mycoplasm genitalium?
Mycoplasm genitalium was selected because it contains the smallest genome of any free-living organism - only 525 genes, as opposed to the 4,288 of E. coli, a more traditional laboratory bacterium. It has been the focus of several recent bioengineering efforts, including the work of the J. Craig Venter Institute's 2008 synthesis of the first artificial chromosome.
"The goal hasn't only been to understand M. genitalium better," said co-first author and Stanford biophysics graduate student Jonathan Karr in a Stanford press release. "It's to understand biology generally."
Advantages of going digital
The purely computational cell opens up procedures that would be difficult to perform in an actual organism, as well as opportunities to reexamine experimental data.
In the paper, the model is used to demonstrate a number of these approaches, including detailed investigations of DNA-binding protein dynamics and the identification of new gene functions.
The program also allowed the researchers to address aspects of cell behavior that emerge from vast numbers of interacting factors.
The researchers had noticed, for instance, that the length of individual stages in the cell cycle varied from cell to cell, while the length of the overall cycle was much more consistent. Consulting the model, the researchers hypothesized that the overall cell cycle's lack of variation was the result of a built-in negative feedback mechanism.
Cells that took longer to begin DNA replication had time to amass a large pool of free nucleotides. The actual replication step, which uses these nucleotides to form new DNA strands, then passed relatively quickly. Cells that went through the initial step quicker, on the other hand, had no nucleotide surplus. Replication ended up slowing to the rate of nucleotide production.
These kinds of findings remain hypotheses until they're confirmed by real-world experiments, but they promise to accelerate the process of scientific inquiry.
"If you use a model to guide your experiments, you're going to discover things faster. We've shown that time and time again," said Covert.
Do you think bio-CAD will truly unleash a revolution in biological engineering?
Image: noegranado