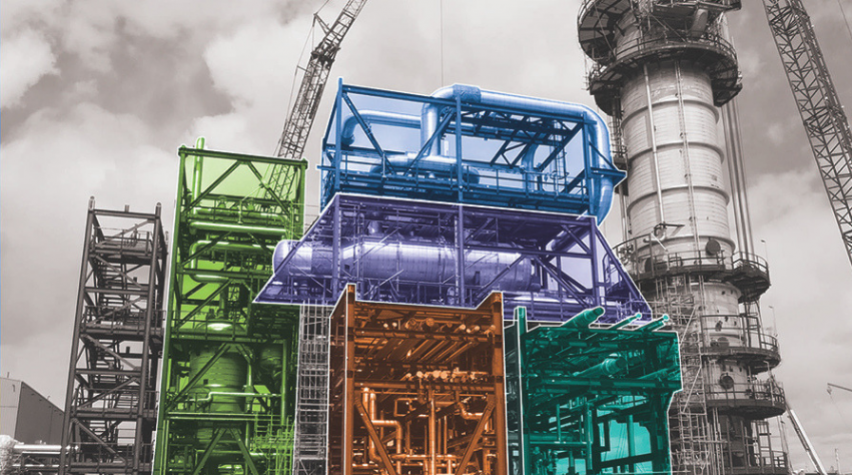
Many advanced-manufacturing topics sit at the interface between traditional disciplines. For example, additive manufacturing draws from manufacturing engineering, mechanical engineering, computer science, and materials science, while smart manufacturing touches on industrial engineering, computer science, and chemical engineering.
In contrast, process intensification (PI) is uniquely tied to the fundamental principles of chemical engineering. In a nutshell, it is the application of the best possible technology solutions to classic problems in transport phenomena, reaction engineering, and separation science. Its definitions are diverse:
- Improvement of a chemical engineering process by adopting innovative technologies and substantially reducing the equipment size, energy consumption, or waste formulation, and lead to cost-effective, safer, sustainable process (Stankiewicz and Moulijn, Chemical Engineering Progress, 96, 22-34, 2000).
- Providing radically innovative principles ("paradigm shift") in process and equipment design which can benefit (often with more than a factor two) process and chain efficiency, capital and operating expenses, quality, wastes, process safety and more (European Roadmap on Process Intensification).
- A paradigm shift in chemistry, process science, and equipment design which proves to be beneficial in reducing operating and capital expenses, process efficiency improvements, waste reduction, and process safety improvements (NSF Process Intensification Workshop, 2014).
The different forms of PI
What ultimately defines PI is the application of fundamental sciences and engineering creativity to develop step-out hardware and operating solutions, removing process bottlenecks and improving capital and operating efficiencies. It takes different forms:
- Rethinking classical hardware solutions, such as using microreactors in place of conventional reaction systems,
- Combining classical unit operations into a single processing step, such as reactive distillation or membrane reactors,
- Applying novel driving forces to create step changes in process effectiveness, such as the use of acoustics or microwaves to drive transport or reactions, or
- Using cyclic operating and control parameters to improve average performance, such as reverse flow reactors or cyclic distillation.
The original paradigm
If PI is so clearly tied to classical chemical engineering fundamentals, one has to ask why it is novel and not the norm. This is linked to the historical success of the original paradigm in chemical processing—unit operations. The concept of unit operations that were capable of carrying out specific transformations (mixing, heating, reaction, separation, etc.) was introduced by George Davis in 1888.
By splitting phenomena into distinct physical pieces of equipment, the application of unit operations allowed for the design and operation of a broad set of process chemistries with a limited amount of computation and modeling horsepower.
It was nearly 100 years later that more mathematically rigorous and fundamental physics-based approaches became the norm for educating chemical engineers. Because classical unit operations were very successful, there wasn’t an impetus to use the more general transport and reaction engineering framework to radically redesign unit operations. The new formalism for chemical engineering was instead used to optimize and better understand the landscape of hardware that already existed.
Feedstock shifts
Today, that has changed. Reassessment of classical unit operations is being driven in part by external pressures for more efficient solutions. One is the rise in new feedstocks that may, or may not, fit within existing process infrastructure. Feedstock shifts include the rise in availability of light gas molecules as feedstock for fuels and chemicals products, as well as the potential opportunity to convert streams that were historically thought of as waste — including waste biomass — into a feedstock.
A second driver is in the rise in expectations around energy efficiency and environmental footprint. This shift can be seen in shifts in regulatory requirements and also as a change in the expectations of businesses and consumers. Finally, the process industries are affected by dynamic shifts in customer demands that are driven by the general acceleration in new technology deployment. A good example is the shift in paper demand, where decline in printed materials is occurring at the same time as the rise in materials to support growth in direct shipping to consumers.
Technology advances that empower PI
At the same time, advances in technologies outside the process industries are creating huge potential for new and innovative solutions. These technology advances are particularly enabling for PI:
- One example is the advances we see in advanced materials developed for applications in batteries and fuel cells. These materials create a new platform of kinetically active materials that are also viable as separation platforms.
- A second area is the further development of novel component manufacturing technologies like generative design coupled with additive manufacturing. Such approaches could enable step-out performance shifts in small-scale reaction and heat exchange systems.
- Finally, the potential rise of very low-cost (albeit intermittent) electricity from renewable sources creates new opportunities to utilize technologies such as microwave heating and electrochemistry in novel ways.
The emergence of RAPID
The RAPID Manufacturing Institute was created in 2016 with the mission of focusing U.S. efforts in PI to transform the process industries. The goal of this transformation is to create a more competitive, operationally and energy-efficient process industry sector in the US. RAPID’s key activities initially focused on building a community among industry, academics, nonprofits, and national labs to foster a common understanding of the specific process needs that could potentially be met by PI solutions.
Their efforts created a PI technology roadmap, highlights of which are publicly available on the RAPID website. Subsequently, RAPID has begun working with its members to create a portfolio of R&D projects and a slate of educational programming that facilitates the broad adoption of new PI technologies.
The portfolio currently includes 31 R&D projects spanning cyclic operation of conventional distillation technologies, novel materials and manufacturing methods for microreactors, shifts in batch to continuous processing for specialty chemicals, and modular conversion technologies for distributed biomass resources. Additionally, RAPID has developed an educational portfolio of webinars and eLearning courses to enable a better understanding of PI across a varied set of constituents from industry members to graduate and undergraduate students.
There is still a great deal of work to accomplish in order to bring the potential of PI to the process industries as a whole. RAPID is working with its member organizations to help the process industries take these first crucial steps.
Comments
Thank you Jim Bielenberg for this great piece on process intensification. My current research is hinged on process intensification; the aspect that pertain to alternative reactor design for methane utilization. I have been thinking of taking this beyond research. So, apart, from having knowledge of a particular field, what other skills do I need to learn to become a consultant in process intensification? Or in simple terms, what is the career path to process intensification? Thank you sir. Kind regards, Ojotule Onoja